Translate this page into:
Pathophysiology and Management of Hypercoagulation in Infectious Diseases
Abhishek Tandayam, MBBS, MD Medical Affairs, Dr Reddy's Laboratories Hyderabad India megaabhi@gmail.com
This article was originally published by Thieme Medical and Scientific Publishers Pvt. Ltd. and was migrated to Scientific Scholar after the change of Publisher.
Abstract
Numerous systemic infections may have hypercoagulation as one of the complications, which may range from asymptomatic presentation of elevation of biochemical markers of coagulation such as that of fibrin and thrombin generation, to a much severe, symptomatic, life-threatening, disseminated intravascular coagulation (DIC), which results in the formation of thrombi in the microvasculature of various organs. This phenomenon contributes to increase in morbidity and mortality in various infectious diseases. The current review discusses various mechanisms of hypercoagulation during infections such as tissue factor activation, endothelial cell activation, inhibition of physiological anticoagulant pathways, and fibrinolysis inhibition. The review also discusses pathophysiological changes in the coagulation system and its management in the recent pandemic of COVID-19. The article also discusses role of various parenteral and oral anticoagulants in the management of infectious diseases. The review provides clinical data on various anticoagulants used during hospitalization and extended prophylaxis for the management of venous thromboembolism in various infections.
Methodology Because this is a review of published literature and no humans or animals were involved, ethical committee approval was not required and patient consent was not required.
Keywords
hypercoagulation
management
pathophysiology
infectious diseases
DIC
COVID-19
anticoagulants
Introduction
In invertebrates (lower organisms), hemostasis and immunity are mediated by the same line of cells, the hemocytes.1 Having characteristic features of both platelets and neutrophils, hemocytes are capable of phagocytosing invading pathogens and undergoing degranulation, releasing numerous immune mediators. Moreover, on activation, hemocytes lead to the ‘clotting’ of hemolymph for either maintaining the hemostasis in the organism or to wall-off and restrict an infection in an attempt to limit the dissemination of pathogens. In a similar manner, coagulation and clotting in higher organisms such as mammals are assumed to play a role in restricting pathogen dissemination and preventing the spread of infection throughout the body via bloodstream.
Numerous systemic infections may have hypercoagulation as one of the complications, which may range from asymptomatic presentation of elevation of biochemical markers of coagulation such as that of fibrin and thrombin generation, to a much severe, symptomatic, life-threatening, disseminated intravascular coagulation (DIC), which results in the formation of thrombi in the microvasculature of various organs.2 Studies have shown that greater than 80% of sepsis patients present with some degree of either subclinical or clinical coagulopathy, and these patients usually have a much greater morbidity and mortality rates.
DIC is a pathological condition, which involves the activation of the systemic clotting cascade leading to fibrin deposition and formation of thrombi throughout the vasculature. This may lead to the formation of pulmonary embolism, multiorgan dysfunction syndrome (MODS), and finally death.3, 4, 5, 6, 7
DIC leads to high morbidity and mortality rates in both bacterial and nonbacterial infections due to multiorgan failure (MOF).8, 9
As of today, there is no specific treatment for DIC other than supportive treatment.10 The only effective therapy must focus only on the management of the underlying pathology such as control of infections.
Although DIC results from hypercoagulation, but due to the consumption of platelets and coagulation factors, it may also paradoxically result in severe bleeding episodes, including hemorrhagic stroke, which are very frequently seen and further complicate the management of this fulminant condition.11
During infections, activated endothelial cells and mononuclear cells are the main source of proinflammatory cytokines, which are responsible for the derangement and disturbances of the coagulation system.12
Both preclinical and clinical studies of gram-negative bacteria infection-induced sepsis have highlighted the pivotal role of proinflammatory cytokines in the exponential activation of the coagulation cascade.13
Coagulation system activation and propagation has also been proved during nonbacterial infections (viral, protozoal, and fungal).14, 15, 16, 17
The activation of the coagulation system and resulting deposition of fibrin as a consequence of inflammation may be regarded as a physiological protective mechanism in constraining the inflammatory activity to the site of infection or injury.18
Current available standard treatments for managing septic shock are generalized, and therefore slightly ineffective for managing coagulopathy induced specifically by infections.19 The above-mentioned generalized management strategies include the use of antibiotics and corticosteroids, oxygen supplementation, and resuscitation of fluid imbalance with the ultimate goal of eliminating the underlying infection and maintaining homeostasis. These strategies usually do not deal with the source of coagulopathy.
Pathophysiologic Mechanisms of Inflammation-Induced Hypercoagulation
Coagulation disturbance due to inflammation is the result of increased formation of fibrin and defective degradation of fibrin leading to diffuse fibrin deposition in the vasculature.5, 20 Increase in fibrin formation is a result of tissue factor (TF)-mediated thrombin formation and simultaneous down regulation of inhibitory coagulation mechanisms, for example, protein C and S systems. The depression of endogenous thrombolysis system is due mainly to an elevated circulating levels of plasminogen activator inhibitor (PAI)-1, the main inhibitor of plasminogen activation.
1. Tissue factor activation
A membrane-bound protein weighing 4.5 kDa is constitutively expressed on various cells all across the body.21 These cells are located mostly in tissues that are not in direct contact with blood, for example, the adventitial layer of larger blood vessels. The complex of TF and the plasma protein coagulation factor VII(a) serve as a initiating factor for the activation of coagulation.
Upon cell surface expression, TF interacts with coagulation factor VII, either in its zymogen or activated form.22 Upon complex formation with TF, factor VII is activated, and the complex leads to the activation of both coagulation factors IX and X.23 Activated factors IXa and Xa catalyze the activation of factors X and II (prothrombin), respectively.
Inducible form of TF is predominantly expressed by monocytes and macrophages. The presence of platelets and granulocytes leads to the expression of TF on monocytes in a P-selectin-dependent reaction.24 This expression may be due to the activation of nuclear factor k B as a result of binding of activated platelets to monocytes and neutrophils.
In addition to the inducible TF, a soluble form of TF is detectable in human plasma. DIC also results in the elevation of soluble TF levels.25
2. Role of endothelial cells
Vascular endothelial cells and innate immune system (mostly mononuclear cells) play a crucial and central role in all mechanisms that lead to inflammation-induced coagulopathy.26
Endothelial cells not only respond to the cytokines released by activated leukocytes but also are capable of releasing cytokines themselves.13 Furthermore, endothelial cells are capable of expressing growth factors and adhesion molecules that not only potentiate the inflammatory response further but may also alter the coagulation response.
Moreover, it has been recently shown that, along with indirect effects, endothelial cells are capable of interfering directly with the initiation and propagation of fibrin formation and degradation during severe infections.27
3. Physiological anticoagulant pathways
Coagulation inhibitors occur naturally in human plasma to keep a control on the activation of coagulation.28
Soluble inhibitors of coagulation include proteins C and S, antithrombin (AT), and tissue factor pathway inhibitor (TFPI).29
AT is one of the most important and potent inhibitors of the activated coagulation cascade, and significantly reduced plasma levels are observed in sepsis.30
In the due course of DIC, the activity of AT may be affected in several ways. First, a decreased protein synthesis and increased clearance lead to decreased absolute levels of the inhibitor.31 Secondly, the activity of the AT may be decreased due to the decreased availability of glycosaminoglycans, which may serve as the physiological heparin-like cofactor of AT.32 The production of glycosaminoglycans by endothelial cells may be decreased because of the presence of circulating cytokines, leading to impaired inhibitory potential of AT.33
Antithrombin also possess an anti-inflammatory effect apart from anticoagulant effect as shown in animal models.34 This gives an additional protective value for the antithrombin.
Protein C and its cofactor protein S are the second line of defense against coagulation.35 Thrombin interacts with the endothelial cell membrane-bound molecule thrombomodulin, and this complex leads to the activation of protein C forming protein Ca (PCa).36 In addition, the thrombin–thrombomodulin complex potentiates the activation of the thrombin activatable fibrinolytic inhibitor (TAFI).37 PCa inhibits coagulation factors Va and VIIIa by proteolytic cleavage, thus slowing down the coagulation cascade.
Endothelial cells, mainly of large vasculature, express an endothelial protein C receptor (EPCR), which potentiates the activation of protein C at the cell surface.38
Activated protein C possess anti-inflammatory effects on granulocytes and mononuclear cells, apart from its usual anticoagulant activity.39
Cell culture experiments have shown that TNFa and IL-1 may significantly decrease the cellular expression of thrombomodulin during conditions associated with systemic inflammation and DIC.40
TFPI constitutes a third inhibitory mechanism.41 Human studies have confirmed the capability of TFPI to block the endotoxin triggered procoagulant activity.42
In general, the presence of normal plasma levels and intact function of inhibitors are important for the defense against DIC.12
4. Fibrinolysis
Experimental studies of severe infections have shown activation of fibrinolysis, as demonstrated by an initial activation of plasminogen, followed by a significant impairment due to the release of PAI-1 in blood.43 PAI 1 strongly inhibits fibrinolysis, shifting a net balance toward procoagulant situation.
Fibrinolytic activity is significantly controlled by PAI-1, the main inhibitor of this system.44
In human studies of DIC, elevated plasma levels of PAI-1 served as one of the best predictors of mortality.45 PAI-1 being an acute-phase protein, a higher plasma concentration of the same may be a marker of disease rather than just a causal factor. Interestingly, platelet α-granules express very large amounts of PAI-1 and release PAI-1 on their activation. Severe infections and inflammation lead to the activation of platelets and later may further elevate the levels of PAI-1and contribute to the shutoff of the fibrinolytic system.
Hyper Coagulation in COVID-19: A Recent Pandemic
Coronavirus disease 2019 (COVID-19) is a respiratory infection caused by a virus, severe acute respiratory syndrome coronavirus 2 (SARS-CoV-2). The most common cause of death is the respiratory failure, but coagulation hyper activation due to excessive immune/inflammatory responses (representing the so-called cytokine storm), vascular thrombosis and DIC, and ultimately multiple-organ failure (MOF) may also serve as causes of death.46 Rapid deterioration occurs in the patients with thrombosis and DIC.
The rate of DIC in COVID-19 infection has been reported to be high (71.4%) for non-survivors and about 0.6% for survivors.47 This proves the fulminant nature of DIC.
The exact mechanisms underlying coagulopathy are not fully understood; however, it is presumed that the dysregulated and exaggerated immune reactions mediated by proinflammatory cytokines, death of lymphocytes, hypoxia, and endothelial cell damage are the causes.48 Bleeding property is less common.
The most marked abnormal coagulation parameter in severe COVID-19 infection is an excessively raised D-dimer levels.47, 49, 50 Initial COVID-19 studies found 46% of all cases demonstrate abnormally raised D-dimer levels.50 In a study of 343 COVID 19 patients, it was found that D-dimer levels of more than 2.0 mg/L served as one of the predictor of mortality with a sensitivity and specificity of 92% and 83%, respectivey.51
In severe COVID-19 infection, plasma levels of pro-inflammatory cytokines, such as interleukin (IL)-1, IL-6, and tumor necrosis factor-α (TNF-α) are markedly elevated.50 IL-6 stimulates tissue factor expression on monocytes and macrophages, which ultimately leads to thrombin formation.
COVID infections are also associated with a significant fibrinolytic profile.52 In human SARS-CoV-1 infection, plasma levels of tissue-type plasminogen activator (t-PA) were shown to be six-fold elevated than normal levels.53 It is most probably that the infection induced inflammation, causing endothelial cell disturbances that resulted in significant release of plasminogen activators, which can explain the high plasma levels of d-dimer in the most severe cases of COVID-19. Also, effects of plasmin on metalloproteinases may result in the modification of the extracellular matrix potentiating capillary leakage and edema of the lungs.
Surprisingly, the effects on activators of plasminogen system do not induce a hyperfibrinolytic state or an higher risk of systemic bleeding events in patients with COVID-19 infection.54 There is a significant relationship between the bronchoalveolar system coagulation and fibrinolysis and the development of acute respiratory distress syndrome (ARDS), in which fibrin deposition in the lungs as a result of disturbed bronchoalveolar fibrin turnover is a crucial step. The clinical and laboratory data of severe ARDS in ventilated COVID-19-infected patients and important abnormalities of the coagulation system may point toward a potential role of the bronchoalveolar fibrin turnover in the severe COVID-19 patients. The clinical picture of the COVID-19 coagulopathy is usually prothrombotic state in the absence of a consumption coagulopathy, which leads to higher rates of thromboembolism (mostly venous and possibly arterial), and not so many hemorrhagic complications. Prolonged immobilization and damage to the vascular system are other causes of increased risk of thrombosis.
Histopathology studies during post-mortem examinations in patients succumbed to COVID-19 have shown typical platelet-rich thrombotic depositions in the microvessels of the lungs along foci of local hemorrhages and entrapment and accumulation of inflammatory cells, such as neutrophils, in the capillaries of alveoli.55 This picture resembles that of pulmonary thrombotic microangiopathy.
Thrombotic microangiopathy results from an increased adhesion of platelets to the vascular endothelium and further platelet activation and aggregation leading to consumptive thrombocytopenia (Fig. 1).56
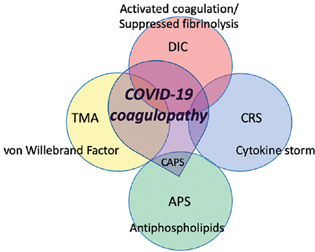
- Pathological characteristics of COVID-19-induced coagulopathy. Source: Adapted from Levi et al.54
A study involving 1,561 patients with COVID-19 by Yu et al also demonstrated a significant rise in coagulation parameters.57 The study showed a 260.00% rise in d-dimer levels in severe disease, with plasma levels ranging from 0.9 to 4.6 µg/mL with a median level of 1.8 µg/mL. In a meta-analysis of 13 cohort studies (including the above study), patients with severe COVID-19 had a rise in the mean value of D-dimer by 0.91 μg/mL (95% confidence interval, 0.51–1.31, p < 0.001) compared to those with non-severe disease, and the risk of severe infection was associated with D-dimer greater than 0.5 μg/mL (odds ratio = 5.78, 95% confidence interval, 2.16–15.44, p < 0.001) on admission.
Guan et al noticed an abnormally elevated d-dimer levels in 46.4% (n =560) cases with prevalence being 43% and 60% in non-severe and severely ill intensive care unit (ICU) patients, respectively.58
A meta-analysis of 14 clinical studies demonstrated that the incidence of COVID-19 patients developing DIC was 3%, and a statistically significant association between DIC and mortality was observed. DIC is associated with the severity and poor prognosis of COVID-19 patients.59 Therefore, careful attention should be given to coagulation abnormalities in COVID-19 patients. Careful monitoring of coagulation parameters may definitely improve the prognosis of COVID-19 patients admitted to a hospital.59
Numerous clinical studies have shown varying incidence rates of venous thromboembolism (VTE) in COVID-19 patients.60 A meta-analysis of studies in COVID-19 in-hospital patients found that the overall VTE prevalence was 14.1%. The VTE prevalence was more in studies that used ultrasound screening as a diagnostic tool (40.3%; 95% CI, 27.0–54.3) compared to studies in which ultrasound was not used (9.5%; 95% CI, 7.5–11.7).
A retrospective analysis involving 2,773 hospitalized COVID-19 patients from a single center in the USA showed in-hospital mortality in 22.5% of patients who received therapeutic anticoagulation and 22.8% of patients with no anticoagulation.61 The study further showed that in a subgroup of 395 patients on mechanical ventilation, 29.1% of the COVID patients who were given anticoagulation and 62.7% of those who were not, died.
Guidelines for Management of Hypercoagability During COVID-19
American Society of Hematology 2021 guidelines on the use of anticoagulation for thromboprophylaxis in COVID-19 patients
Recommendation 1. The ASH guideline panel suggests using prophylactic-intensity over intermediate-intensity or therapeutic intensity anticoagulation for patients with COVID-19-related critical illness who do not have suspected or confirmed VTE (conditional recommendation based on very low certainty in the evidence about effects).62
Recommendation 2. The ASH guideline panel suggests using prophylactic-intensity over intermediate-intensity or therapeutic intensity anticoagulation for patients with COVID-19-related acute illness who do not have suspected or confirmed VTE (conditional recommendation based on very low certainty in the evidence about effects).
Hypercoagability in Tuberculosis
Tuberculosis is an infectious disease caused by the bacteria Mycobacterium tuberculosis. One-third of the population worldwide is estimated to be infected with the tuberculosis bacterium. The lungs are primarily infected in approximately 90% of cases, while the genitourinary tract, gastrointestinal tract, lymph nodes, bones, muscles, and the central nervous system can also be affected in the so-called disease extrapulmonary form of tuberculosis. It is a well-known fact that a hypercoagulable state associated with tuberculosis may cause thromboembolism, and this complication is found in about 0.6 to 1.0% of tuberculosis patients. Further, potential drug–drug interactions may occur between rifampicin, the mainstay of anti-tuberculosis drugs, and warfarin, which is commonly used to treat thromboembolism.63
Parenteral Anticoagulants for Prophylaxis of Thromboembolism in Hospitalized Acutely Ill Medical Patients
Venous thromboembolism (VTE) is a very common disease that is seen in hospitalized surgical and medical patients.64
A wide variety of parenteral anticoagulants are routinely available and recommended for the prevention and treatment of VTE, including unfractionated heparin (UFH), low-molecular-weight heparins (enoxaparin, dalteparin, and tinzaparin), and new class of highly selective anti-Xa inhibitors (fondaparinux).65
UFH was a traditional anticoagulant used from a long time in the prevention and treatment of VTE.65 Thromboprophylaxis with UFH is very effective in preventing VTE episodes and in treatment of VTE, but is unfortunately associated with a high risk of bleeding complications. UFH use is also restricted due to the necessity of regular monitoring of coagulation parameters and dosage adjustments. Over the last decade, LMWHs have replaced UFH as the standard anticoagulant in VTE prevention and treatment. More recently, highly selective factor Xa inhibitor, synthetic pentasaccharide fondaparinux has been developed and approved by the Food and Drug Administration (FDA).
The FDA has approved the use of enoxaparin and dalteparin for parenteral anticoagulation for prophylaxis of VTE in acutely ill hospitalized patients.65
Meta-analyses of numerous randomized clinical studies have proven that the LMWHs are at least as efficacious and safe as UFH in the prophylaxis of VTE in both medical and surgical hospitalized patients.66 In hospitalized medical patients, a meta-analysis of nine clinical trials including data from 4,669 patients demonstrated that both LMWHs and UFH were equally effective in reducing the incidences of VTE, but LMWHs were more safer in that they reduced bleeding complications by 52% compared to UFH (p = 0.049).
The MEDINOX trial showed that in acutely ill hospitalized medical patients, the incidence of symptomatic or asymptomatic VTE was reduced with enoxaparin 40 mg once daily compared with placebo (5.5% vs. 14.9%; p < 0.001) with no statistically significant increase in major bleeding events (3.4% vs. 2.0%).67
Enoxaparin 40 mg SC once daily was equally safe and efficacious as UFH 5000U TID in other clinical studies.68, 69
In patients presenting with acute ischemic stroke, the risk of symptomatic or non-symptomatic VTE with enoxaparin 40 mg SC once daily was reduced compared with UFH 5,000 U bid (10% vs. 18%; p = 0.001), without increasing the risk of symptomatic intracranial bleeding events (1% vs. 1%; p = 0.55) but an increased incidence of major extracranial bleeding events (1% vs. 0; p = 0.015).70
The PREVENT trial showed that in acutely ill medical patients, dalteparin 5,000 IU reduced the rate of symptomatic or non-symptomatic VTE compared with placebo (2.77% vs. 4.96%; p = 0.0015) with no statistically significant increase in major bleeding events (0.49% vs. 0.16%; p = 0.15).71
The ARTEMIS trial showed in acutely ill medical patients who are at risk of VTE, fondaparinux reduced the incidence of asymptomatic or symptomatic VTE compared with the placebo (5.6% vs. 10.5%; p = 0.029) without increasing the rates of major bleeding (0.2% vs. 0.2%).72
Extended thromboprophylaxis with parenteral anticoagulants in acutely ill medical patients was studied in the EXCLAIM trial, in which subjects were randomized to enoxaparin 40 mg SC once daily or placebo for 28 ± 4 days after receiving in hospital enoxaparin for 10 ± 4 days.73 Extended thromboprophylaxis reduced VTE incidences compared with placebo (2.5% vs. 4.0% respectively; absolute risk difference-1.53%) but unfortunately at the expense of significantly higher major bleeding events (0.8% vs. 0.3%, respectively; absolute risk difference 0.51%). Adverse events leading to death were similar between the two study groups (1.3% vs.1.5%). In conclusion, results proved that the benefit of extended thromboprophylaxis with enoxaparin outweighed the risk of bleeding not in the overall population but only in a selected subgroups, including patients who were immobilized, women and those aged > 75 years.
Oral Anticoagulation in Infectious Diseases
Direct oral anticoagulants (DOACs) have rapidly become attractive alternatives to the conventional standard of care in anticoagulation, i.e., vitamin K antagonists.74
DOACs such as rivaroxaban, dabigatran, apixaban, edoxaban, and betrixaban are used in several cardiovascular conditions for the prevention of thromboembolisms.75
DOACS have many advantages compared with conventional VKAs including fewer coagulation monitoring requirements, lesser frequent follow-ups, faster drug onset and offset effects (vital for peri procedural and reversal of acute bleeding), and fewer drug– drug and food interactions.76
Rivaroxaban and betrixaban are the two DOACs approved by the FDA for the prophylaxis of VTE in acutely ill medical patients.74
The ADOPT trial that was a double-blind, double-dummy, randomized, placebo-controlled trial involving 6,528 acutely ill medical patients, compared apixaban 2.5 mg twice daily for 30 days with enoxaparin 40 mg SC once daily up to 14 days followed by oral placebo for 30 days.77 Results demonstrated that no significant difference in the primary efficacy endpoint of VTE at 30 days (2.71% in apixaban group vs. 3.06% in enoxaparin group; HR 0.87 [0.62–1.23; p = 0.44). Major bleeding during treatment period of 30 days was significantly higher with apixaban (0.47% in apixaban group vs. 0.19% in enoxaparin group; RR 2.58 [1.02–7.24]; p = 0.04). No significant difference was seen in mortality between the two groups (4.1% in each group).
In a multicenter, randomized, double-blind trial (MAGELLIAN) involving 8,101 patients, 40 years of age or older who were hospitalized for an acute medical illness and received either subcutaneous enoxaparin, 40 mg once daily, for 10 ± 4 days and oral placebo for 35 ± 4 days or subcutaneous placebo for 10 ± 4 days and oral rivaroxaban, 10 mg once daily, for 35 ± 4 days, showed rivaroxaban was at least noninferior to enoxaparin for standard duration thromboprophylaxis (2.7% vs. 2.7%, p = 0.03 for non-inferiority).78 Extended-duration rivaroxaban reduced the risk of venous thromboembolism (4.4% vs. 5.7%, p = 0.02). Rivaroxaban was associated with an increased risk of bleeding both at the end of standard duration prophylaxis (2.8% vs. 1.2%, p < 0.001) and extended prophylaxis (4.1% vs. 1.7%, p < 0.001).
The dose of rivaroxaban in acutely ill medical patients is 10 mg once daily, with or without food, in hospital and after hospital discharge for a total recommended duration of 31 to 39 days in patients with creatinine clearance ≥ 15 mL/min.79 However, it is contraindicated in patients with creatinine clearance < 15 mL/min.
The APEX (Acute Medically Ill VTE [venous thromboembolism] Prevention with Extended Duration Betrixaban) trial, which was a randomized, active-controlled, double-blind, double-dummy, multinational trial, involving 7,513 patients (40 years of age or older and had risk factors for VTE) who were randomly assigned to receive subcutaneous enoxaparin (at a dose of 40 mg once daily) for 10 ± 4 days plus oral placebo for 35 to 42 days or subcutaneous placebo for 10 ± 4 days plus oral betrixaban (at a dose of 80 mg once daily) for 35 to 42 days. The primary efficacy outcome was a composite of asymptomatic proximal deep vein thrombosis and symptomatic venous thromboembolism and the principal safety outcome was major bleeding. A sequential analyses was performed in three prespecified cohorts, cohort 1 including patients who had elevated d-dimer levels, cohort 2 including patients who had elevated d-dimer levels or an aged ≥ 75 years, and cohort 3 including all the enrolled patients.80 In cohort 1, no significant differences was seen between the two treatment groups (6.9% in the betrixaban group and 8.5% in the enoxaparin group [0.81; 95% CI, 0.65–1.00; p = 0.054]). The exploratory analysis in the other two cohorts showed benefits with betrixaban with rates of primary outcomes being 5.6% and 7.1%, respectively, in cohort 2 (relative risk, 0.80; 95% CI, 0.66–0.98; p = 0.03) and 5.3% and 7.0%, respectively, in cohort 3 (relative risk, 0.76; 95% CI, 0.63–0.92; p = 0.006). In terms of safety events, major events was not significantly different between the groups (0.7% vs. 0.6%, odds ratio, 1.19; 95% CI, 0.67–2.12; p = 0.55). No significant in fatal bleeding was seen with betrixaban. The incidences of clinically relevant non-major bleeding was, however, higher with betrixaban in all cohorts. The net clinical benefit, which is a composite of any primary efficacy end point or principal safety outcome), was seen in 5.8% of the betrixaban group and 7.3% of the enoxaparin group (HR, 0.78; 95% CI, 0.65–0.95; p = 0.01). In the exploratory analysis using more specific and reliable d-dimer testing, which is used in the central laboratory, benefit for betrixaban was seen in all cohorts including cohort 1.
The MARINER trial was another trial of extended thromboprophylaxis, which was a double-blind, randomized trial involving around 12,024 medically ill patients who were at a high risk for VTE based on a modified International Medical Prevention Registry on Venous Thromboembolism (IMPROVE) score of ≥ 4 or a score of 2 or 3 in addition with a plasma d-dimer levels of higher than two times the upper limit of the normal range.81 Patients were randomly assigned at hospital discharge to either rivaroxaban 10 mg orally once-daily or placebo orally for 45 days. The primary efficacy outcome, which was the composite of symptomatic VTE or death due to VTE, was not significantly different between the groups (0.83% vs. 1.10% respectively, HR, 0.76, p = 0.14). The incidence of major bleeding was low in both groups (0.28% vs. 0.15% respectively. Though the MARINER trial did not demonstrate an overall statistically significant difference between rivaroxaban and placebo in reducing VTE-related death 45 days after hospital discharge, this strategy of extended thromboprophylaxis with rivaroxaban reduced the incidence of symptomatic VTE by 56% with no much increase in the rates of major bleeding.
Anticoagulation in COVID-19
The risk of VTE ranges from 4.4 to 8.2% among the hospitalized patients with COVID-19.82 The risk may increase up to 53.8%, among severely ill patients with COVID-19 pneumonia admitted to an intensive care unit (ICU). The World Health Organization recommends the use of pharmacological thromboprophylaxis with heparin in COVID-19 patients to prevent the occurrence of VTE. The use of heparins has shown to reduce morbidity and mortality in hospitalized patients with severe COVID-19 disease, probably because it has anti-inflammatory and antiviral proprieties. The once daily dosing regimen of low-molecular-weight heparins or fondaparinux can be preferred over unfractionated heparin to reduce the exposure of healthcare workers to active cases of COVID-19 and personal protective equipment usage.
Albania et al conducted a cohort study among COVID-19 patients with 799 (57%) patients who received enoxaparin at least once during the hospitalization of 604 (43%) patients who were not included in the control cohort.83 This study concluded that treatment with enoxaparin during hospital stay is associated with a lower death rate and, while results from randomized clinical trials were still awaiting, this study supported the use of enoxaparin as thromboprophylaxis in all patients admitted for COVID-19. Moreover, when enoxaparin is used on the wards, it reduces the risk of admissions to ICU.
Initial observational cohort clinical studies demonstrated high rates of VTE in critically ill COVID-19 patients despite the use of standard conventional prophylactic doses of heparin-based anticoagulant regimens.84 Moreover, autopsy data that was published demonstrated microthrombi in multiple organs.85
A retrospective cohort study among patients with SARS-CoV2 during the Italian outbreak from February 18 to April 30, 2020, to evaluate the antithrombotic and anti-inflammatory effects of fondaparinux and enoxaparin according to particular laboratory markers found no significant differences between the groups in terms of symptomatic VTE. Results also showed that anti-inflammatory effects of fondaparinux and enoxaparin after 3 weeks of prophylactic treatment were similar.86
A multicenter Italian retrospective study involving 186 consecutive symptomatic patients with COVID-19 admitted to five Italian hospitals from February 15 to March 15, 2020, showed that there were no significant differences between fondaparinux and enoxaparin in terms of both symptomatic VTE and major bleeding although fondaparinux had a significant net clinical benefit.82
Benefits of Fondaparinux Over Enoxaparin
The pentasaccharide fondaparinux is the first in class of synthetic antithrombotic agents that acts through highly specific inhibition of factor Xa, devoid of any direct activity against factor IIa.87 This inhibition of factor Xa via antithrombin results in effective inhibition of thrombin generation. Fondaparinux sodium has 100% bioavailability when administered subcutaneously and does not undergo metabolism. Fondaparinux exhibits a linear pharmacokinetic profile in healthy volunteers, with little inter individual variability. The half maximal plasma concentration is reached within 25 minutes, and the dose-independent elimination half-life is 15 hours, allowing once-daily administration.
DOACS in Hospitalized COVID Patients
DOACs usually undergo P-glycoprotein and/or cytochrome P450 (CYP)-based metabolism.88 Numerous classes of drugs lead to drug–drug interactions and modifying the DOAC pharmacokinetic and pharmacodynamic profiles and causing a significant decrease or increase of their anticoagulant effects. In fact, antiviral drugs such as remdesivir are substrates of CYP 3A4-, CYP 2D6-, and CYP 2C8-based metabolism. Dexamethasone, which is used in COVID-19, is also an inducer of CYP3A4.
The numerous drug–drug interactions (antiviral, antibiotics, antihypertensive, bronchodilators, and immunosuppressive drugs), in addition to altered metabolic states that are induced by the acute phase of the disease, can cause an unstable and unpredictable DOAC anticoagulant effect, leading to the risk of thrombotic complications or uncontrolled bleeding.89
In addition, acute kidney injury in hospitalized patients with COVID-19 infection is a frequent finding, with a rate of about 3% to 15% that may increase up to 50% in very severe patients such as those requiring ICU admission.90
The immune response, which produces IL-6 as one of the important mediators, is a characteristic feature of COVID-19 pathogenesis.91 This suggests that COVID-19 disease itself has an impact on the CYP regulation.
In patients admitted to hospital with COVID-19 using DOACs previously due to different indication, DOACs should be discontinued and switched over to an alternative anticoagulant in patients, who are candidates for antiviral drugs or anti-IL6/IL1 therapy.88 The same goes true for the concomitant use of dexamethasone. As the pharmacological effect of dexamethasone on the CYP system may last for a week, DOACs might be restarted again after discharge with a minimum gap of 1 week after stopping dexamethasone.
As the potential risk of interactions between drugs differs with the type of DOAC used, it seems that dabigatran may be the DOAC of choice because it has the lowest risk to interact with COVID-19 drugs that are metabolized through cytochrome P450.92
A retrospective cohort study that acted as a simulation intention-to-treat trial on the effect of anticoagulation therapy in the first 48 hours of hospitalization in 3,625 in patients with COVID.93 A significant reduction in mortality with prophylactic use of apixaban (OR = 0.46, p = 0.001) and enoxaparin (OR = 0.49, p = 0.001) was seen. Therapeutic doses of apixaban also resulted in decreased mortality (OR = 0.57, p = 0.006) but no more benefit compared to prophylactic use.
Action trial, which was an open-label but with blinded adjudication, randomized, multicenter, controlled trial, at 31 sites in Brazil, involving patients (aged ≥ 18 years) hospitalized with COVID-19 and elevated D-dimer concentration, showed in-hospital therapeutic anticoagulation with rivaroxaban (20 mg or 15 mg orally once daily) or enoxaparin (1 mg/kg SC twice daily) followed by rivaroxaban to day 30 did not improve clinical outcomes and increased bleeding when compared to prophylactic anticoagulation.94
Extended thromboprophylaxis for COVID-19 patients after hospital discharge was studied in the MICHELLE trial, which was an open-label, randomized, multicenter trial conducted in Brazil.95 Around 320 COVID-19 patients who were at an increased risk for VTE based on IMPROVE (International Medical Prevention Registry on Venous Thromboembolism venous thromboembolism) score of ≥ 4 or 2–3 with d-dimer levels more than 500 ng/mL) were randomly assigned at hospital discharge, to receive rivaroxaban 10 mg/day or no anticoagulation for 35 days. The primary efficacy endpoint in an intention-to-treat analysis, which was a composite of symptomatic or fatal venous thromboembolism, asymptomatic venous thromboembolism (on bilateral lower-limb venous ultrasonography and CT pulmonary angiogram), symptomatic arterial thromboembolism, and cardiovascular mortality at day 35, was significantly lower in the rivaroxaban group compared with no anticoagulation (3% vs. 9%, OR, 0.33, 95% CI,·0.12–0.90; p = 0·0293). No major bleedings were reported in either of the groups and clinically relevant non-major bleeding events, other bleeding events did not differ significantly between the groups.
Other ongoing clinical studies that are evaluating extended thromboprophylaxis in patients with COVID-19 include a Mexican study in the process of enrolling 130 patients to evaluate prophylactic and therapeutic dose of heparin administered in-hospital followed by rivaroxaban 10 mg once daily or no intervention (NCT04508439). It will evaluate out-of-hospital adverse events and changes in biomarkers. Another study, named XACT trial, will evaluate 150 COVID-19 patients randomized to in-hospital enoxaparin or oral rivaroxaban (10 mg/day or 15 mg/day or 20 mg/day) through discharge for 28 days and the primary endpoint is a combination of death or 30-day all-cause mortality, mechanical ventilation, intubation, or transfer to an ICU (NCT04640181). A much larger trial, ACTIV-4c (NCT04650087), is planning to enroll 4,000 COVID-19 patients in the USA to study the efficacy and safety profile of anticoagulants and antiplatelets (apixaban, aspirin, or placebo) administered to patients who were recovered and discharged from the hospital. The primary aim is to reduce myocardial infarction, stroke, arterial and venous thrombosis, and death within 30 days post hospital discharge for moderate and severe COVID-19. Lastly, the HEAL-COVID (NCT04801940) trial is planning to enroll patients at hospital discharge after a first time admission due to COVID-19 and they will be randomized into three groups (apixaban, atorvastatin, and standard of care). The primary efficacy outcome is hospital-free survival over the next 12 months.
Conflicts of Interest
All authors are the employees of Dr. Reddy's Laboratories, Hyderabad, which is currently marketing some of the parenteral and oral anticoagulants.
Funding None.
References
- Pathogenesis of disseminated intravascular coagulation in sepsis. JAMA. 1993;270(8):975-979.
- [Google Scholar]
- Liver perfusion in sepsis, septic shock, and multiorgan failure. Anat Rec (Hoboken). 2008;291(6):714-720.
- [Google Scholar]
- Sepsis-associated disseminated intravascular coagulation and thromboembolic disease. Mediterr J Hematol Infect Dis. 2010;2(3):e2010024.
- [Google Scholar]
- Methicillin-resistant Staphylococcus aureus bacteremia at a university hospital in Japan. J Infect Chemother. 2012;18(6):841-847.
- [Google Scholar]
- Laboratory markers of coagulation and fibrinolysis.Hemostasis and Thrombosis: Basic Principles and Clinical Practice, 3rd ed. Philadelphia: JB Lippincott; 1994. p. :1197-1210. In: eds.
- [Google Scholar]
- Disseminated intravascular coagulation: pathophysiology, diagnosis, and treatment. New Horiz. 1993;1(2):312-323.
- [Google Scholar]
- Review: infectious diseases and coagulation disorders. J Infect Dis. 1999;180(1):176-186.
- [Google Scholar]
- Diffuse cerebral intravascular coagulation and cerebral infarction in pneumococcal meningitis. Neurocrit Care. 2010;13(2):217-227.
- [Google Scholar]
- Disseminated intravascular coagulation in infectious disease. Semin Thromb Hemost. 2010;36(4):367-377. . © Thieme Medical Publishers
- [Google Scholar]
- Cytokines: triggers of clinical thrombotic disease. Thromb Haemost. 1997;78(1):415-419.
- [Google Scholar]
- Hemostatic defects in dengue hemorrhagic fever. Rev Infect Dis. 1989;11(04):S826-S829.
- [Google Scholar]
- Early markers of blood coagulation and fibrinolysis activation in Argentine hemorrhagic fever. Thromb Haemost. 1995;73(3):368-373.
- [Google Scholar]
- Activation of the coagulation cascade in severe falciparum malaria through the intrinsic pathway. Br J Haematol. 1994;87(1):100-105.
- [Google Scholar]
- Fibrinolysis, inhibitors of blood coagulation, and monocyte derived coagulant activity in acute malaria. Am J Hematol. 1997;54(1):23-29.
- [Google Scholar]
- New diagnostic strategy for sepsis-induced disseminated intravascular coagulation: a prospective single-center observational study. Crit Care. 2014;18(1):R19.
- [Google Scholar]
- Structural biology of tissue factor, the initiator of thrombogenesis in vivo. FASEB J. 1994;8(6):385-390.
- [Google Scholar]
- The crystal structure of the complex of blood coagulation factor VIIa with soluble tissue factor. Nature. 1996;380:41-46. (6569):
- [Google Scholar]
- The activation of factor X and prothrombin by recombinant factor VIIa in vivo is mediated by tissue factor. J Clin Invest. 1993;92(3):1207-1212.
- [Google Scholar]
- Tissue factor expression by monocytes: regulation and pathophysiological roles. Blood Coagulation & Fibrinolysis. 1998;9:S9-14.
- [Google Scholar]
- Determination of plasma tissue factor antigen and its clinical significance. Br J Haematol. 1994;87(2):343-347.
- [Google Scholar]
- Recent insights into the pathogenesis of bacterial sepsis. Neth J Med. 2010;68(4):147-152.
- [Google Scholar]
- Endothelium: interface between coagulation and inflammation. Crit Care Med. 2002;30:S220-S224. (5, Suppl)
- [Google Scholar]
- Sepsis, coagulation, and antithrombin: old lessons and new insights. Semin Thromb Hemost. 2008;34(8):742-746. . © Thieme Medical Publishers
- [Google Scholar]
- Biochemistry and physiology of blood coagulation. Thromb Haemost. 1999;82(2):165-174.
- [Google Scholar]
- Factor VIIa and antithrombin III activity during severe sepsis and septic shock in neutropenic patients. Blood. 1996;88(3):881-886.
- [Google Scholar]
- Effect of human granulocytic elastase on isolated human antithrombin III. Hoppe Seylers Z Physiol Chem. 1981;362(2):103-112.
- [Google Scholar]
- Glycosaminoglycans and the regulation of blood coagulation. Biochem J. 1993;289:313-330. (Pt 2):
- [Google Scholar]
- Human recombinant interleukin-1 beta- and tumor necrosis factor alpha-mediated suppression of heparin-like compounds on cultured porcine aortic endothelial cells. J Cell Physiol. 1990;144(3):383-390.
- [Google Scholar]
- DEGR-factor Xa blocks disseminated intravascular coagulation initiated by Escherichia coli without preventing shock or organ damage. Blood. 1991;78:364-368.
- [Google Scholar]
- Activated protein C in sepsis: a critical review. Curr Opin Hematol. 2008;15(5):481-486.
- [Google Scholar]
- The roles of protein C and thrombomodulin in the regulation of blood coagulation. J Biol Chem. 1989;264(9):4743-4746.
- [Google Scholar]
- Fibrinolysis and the contact system: a role for factor XI in the down-regulation of fibrinolysis. Thromb Haemost. 1999;82(2):243-250.
- [Google Scholar]
- The anticoagulants protein C and protein S display potent antiinflammatory and immunosuppressive effects relevant to transplant biology and therapy. Transplant Proc. 1992;24(5):2302-2303.
- [Google Scholar]
- von Willebrand factor-cleaving protease in thrombotic thrombocytopenic purpura and the hemolytic-uremic syndrome. N Engl J Med. 1998;339(22):1578-1584.
- [Google Scholar]
- Attenuation of tissue thrombosis and hemorrhage by ala-TFPI does not account for its protection against E. coli–a comparative study of treated and untreated non-surviving baboons challenged with LD100 E. coli. Thromb Haemost. 1998;79(5):1048-1053.
- [Google Scholar]
- Tissue factor pathway inhibitor dose-dependently inhibits coagulation activation without influencing the fibrinolytic and cytokine response during human endotoxemia. Blood. 2000;95(4):1124-1129.
- [Google Scholar]
- Apolipoprotein(a) attenuates endogenous fibrinolysis in the rabbit jugular vein thrombosis model in vivo. Circulation. 1997;96(5):1612-1615.
- [Google Scholar]
- 4G/5G promoter polymorphism in the plasminogen-activator-inhibitor-1 gene and outcome of meningococcal disease. Lancet. 1999;354:556-560. (9178):
- [Google Scholar]
- Increase of plasminogen activator inhibitor levels predicts outcome of leukocytopenic patients with sepsis. Thromb Haemost. 1996;75(6):902-907.
- [Google Scholar]
- COVID-19-associated coagulopathy and disseminated intravascular coagulation. Int J Hematol. 2021;113(1):45-57.
- [Google Scholar]
- Abnormal coagulation parameters are associated with poor prognosis in patients with novel coronavirus pneumonia. J Thromb Haemost. 2020;18(4):844-847.
- [Google Scholar]
- Clinical course and risk factors for mortality of adult inpatients with COVID-19 in Wuhan, China: a retrospective cohort study. Lancet. 2020;395:1054-1062. (10229):
- [Google Scholar]
- Clinical features of patients infected with 2019 novel coronavirus in Wuhan, China. Lancet. 2020;395:497-506. (10223):
- [Google Scholar]
- D-dimer levels on admission to predict in-hospital mortality in patients with Covid-19. J Thromb Haemost. 2020;18(6):1324-1329.
- [Google Scholar]
- Mechanisms of severe acute respiratory syndrome coronavirus-induced acute lung injury. MBio. 2013;4(4):e00271-e13.
- [Google Scholar]
- Elevated plasma tissue-type plasminogen activator (t-PA) and soluble thrombomodulin in patients suffering from severe acute respiratory syndrome (SARS) as a possible index for prognosis and treatment strategy. Biomed Environ Sci. 2005;18(4):260-264.
- [Google Scholar]
- COVID-19 coagulopathy: is it disseminated intravascular coagulation? Intern Emerg Med. 2021;16(2):309-312.
- [Google Scholar]
- Pulmonary and cardiac pathology in African American patients with COVID-19: an autopsy series from New Orleans. Lancet Respir Med. 2020;8(7):681-686.
- [Google Scholar]
- D-dimer level is associated with the severity of COVID-19. Thromb Res. 2020;195:219-225.
- [Google Scholar]
- Clinical characteristics of coronavirus disease 2019 in China. N Engl J Med. 2020;382(18):1708-1720.
- [Google Scholar]
- Incidence and impact of disseminated intravascular coagulation in COVID-19 a systematic review and meta-analysis. Thromb Res. 2021;201:23-29.
- [Google Scholar]
- Risk of venous thromboembolism in patients with COVID-19: A systematic review and meta-analysis. Res Pract Thromb Haemost. 2020;4(7):1178-1191.
- [Google Scholar]
- ECR 2020 Book of Abstracts. Insights into Imaging. Abstracts presented at: ECR, Vienna, Austria, March 11–March 15, 2020;11(S1)
- [Google Scholar]
- American Society of Hematology 2021 guidelines on the use of anticoagulation for thromboprophylaxis in patients with COVID-19. Blood Adv. 2021;5(3):872-888.
- [Google Scholar]
- Thromboembolism in mycobacterium tuberculosis infection: analysis and literature review. Infect Chemother. 2019;51(2):142-149.
- [Google Scholar]
- Relative impact of risk factors for deep vein thrombosis and pulmonary embolism: a population-based study. Arch Intern Med. 2002;162(11):1245-1248.
- [Google Scholar]
- Differentiation of parenteral anticoagulants in the prevention and treatment of venous thromboembolism. Thromb J. 2011;9(1):5.
- [CrossRef] [Google Scholar]
- Prevention of venous thromboembolism in internal medicine with unfractionated or low-molecular-weight heparins: a meta-analysis of randomised clinical trials. Thromb Haemost. 2000;83(1):14-19.
- [Google Scholar]
- A comparison of enoxaparin with placebo for the prevention of venous thromboembolism in acutely ill medical patients. N Engl J Med. 1999;341(11):793-800.
- [Google Scholar]
- The venous thrombotic risk in non-surgical patients: epidemiological data and efficacy/safety profile of a low-molecular-weight heparin (enoxaparin) Haemostasis. 1996;26(02):49-56.
- [Google Scholar]
- Randomized comparison of enoxaparin with unfractionated heparin for the prevention of venous thromboembolism in medical patients with heart failure or severe respiratory disease. Am Heart J. 2003;145(4):614-621.
- [Google Scholar]
- The efficacy and safety of enoxaparin versus unfractionated heparin for the prevention of venous thromboembolism after acute ischaemic stroke (PREVAIL Study): an open-label randomised comparison. Lancet. 2007;369:1347-1355. (9570):
- [Google Scholar]
- Randomized, placebo-controlled trial of dalteparin for the prevention of venous thromboembolism in acutely ill medical patients. Circulation. 2004;110(7):874-879.
- [Google Scholar]
- Efficacy and safety of fondaparinux for the prevention of venous thromboembolism in older acute medical patients: randomised placebo controlled trial. BMJ. 2006;332:325-329. (7537):
- [Google Scholar]
- Extended-duration venous thromboembolism prophylaxis in acutely ill medical patients with recently reduced mobility: a randomized trial. Ann Intern Med. 2010;153(1):8-18.
- [Google Scholar]
- Direct oral anticoagulant use: a practical guide to common clinical challenges. J Am Heart Assoc. 2020;9(13):e017559.
- [Google Scholar]
- Evidence gaps in the era of non–vitamin K Oral anticoagulants. J Am Heart Assoc. 2018;7(3):e007338.
- [Google Scholar]
- Direct oral anticoagulant agents: pharmacologic profile, indications, coagulation monitoring, and reversal agents. J Stroke Cerebrovasc Dis. 2018;27(8):2049-2058.
- [Google Scholar]
- Apixaban versus enoxaparin for thromboprophylaxis in medically ill patients. N Engl J Med. 2011;365(23):2167-2177.
- [Google Scholar]
- Rivaroxaban for thromboprophylaxis in acutely ill medical patients. N Engl J Med. 2013;368(6):513-523.
- [Google Scholar]
- 4. XARELTO (rivaroxaban) tablets label. . Accessed September 8, 2022, at:
- [Publisher] [Google Scholar]
- Extended thromboprophylaxis with betrixaban in acutely ill medical patients. N Engl J Med. 2016;375(6):534-544.
- [Google Scholar]
- Rivaroxaban for thromboprophylaxis after hospitalization for medical illness. N Engl J Med. 2018;379(12):1118-1127.
- [Google Scholar]
- Thromboprofilaxys with fondaparinux vs. enoxaparin in hospitalized COVID-19 patients: a multicenter Italian observational study. Front Med (Lausanne). 2020;7:569567.
- [CrossRef] [Google Scholar]
- Thromboprophylaxis with enoxaparin is associated with a lower death rate in patients hospitalized with SARS-CoV-2 infection. A cohort study. EClinicalMedicine. 2020;27:100562.
- [Google Scholar]
- High risk of thrombosis in patients with severe SARS-CoV-2 infection: a multicenter prospective cohort study. Intensive Care Med. 2020;46(6):1089-1098.
- [Google Scholar]
- Postmortem examination of COVID-19 patients reveals diffuse alveolar damage with severe capillary congestion and variegated findings in lungs and other organs suggesting vascular dysfunction. Histopathology. 2020;77(2):198-209.
- [Google Scholar]
- Antithrombotic and anti-inflammatory effects of Fondaparinux and Enoxaparin in hospitalized COVID-19 patients: the Fondenoxavid study. J Blood Med. 2021;12:69-75.
- [Google Scholar]
- Fondaparinux vs enoxaparin for the prevention of venous thromboembolism in major orthopedic surgery: a meta-analysis of 4 randomized double-blind studies. Arch Intern Med. 2002;162(16):1833-1840.
- [Google Scholar]
- Switch from oral anticoagulants to parenteral heparin in SARS-CoV-2 hospitalized patients. Intern Emerg Med. 2020;15(5):751-753.
- [Google Scholar]
- COVID-19 and renal failure: challenges in the delivery of renal replacement therapy. J Clin Med Res. 2020;12(5):276-285.
- [Google Scholar]
- Cytochrome P450-mediated drug interactions in COVID-19 patients: current findings and possible mechanisms. Med Hypotheses. 2020;144:110033.
- [Google Scholar]
- Dabigatran, the oral anticoagulant of choice at discharge in patients with non-valvular atrial fibrillation and COVID-19 infection: the ANIBAL protocol. Drugs Context. 2020;9:9.
- [Google Scholar]
- Anticoagulation in COVID-19: effect of enoxaparin, heparin, and apixaban on mortality. Thromb Haemost. 2020;120(12):1691-1699.
- [Google Scholar]
- Therapeutic versus prophylactic anticoagulation for patients admitted to hospital with COVID-19 and elevated D-dimer concentration (ACTION): an open-label, multicentre, randomised, controlled trial. Lancet. 2021;397:2253-2263. (10291):
- [Google Scholar]
- Rivaroxaban versus no anticoagulation for post-discharge thromboprophylaxis after hospitalisation for COVID-19 (MICHELLE): an open-label, multicentre, randomised, controlled trial. Lancet. 2022;399:50-59. (10319):
- [Google Scholar]