Translate this page into:
Coronavirus and Homo Sapiens in Coronavirus Disease 2019 (COVID-19)
Muralidhar Kanchi, MD, FIACTA, FICA, MBA, FASE Department of Anaesthesiology and Critical Care, Narayana Institute of Cardiac Sciences Narayana Hrudayalaya, Bommasandra Industrial Area, Anekal Taluk, Bangalore 560 099, Karnataka India muralidhar.kanchi.dr@narayanahealth.org
This article was originally published by Thieme Medical and Scientific Publishers Pvt. Ltd. and was migrated to Scientific Scholar after the change of Publisher.
Abstract
The Spanish influenza pandemic of 1918 globally claimed between 50 and 100 million lives. In India, it was referred to as “The Bombay Fever” and accounted for a fifth of the global death toll. The current outbreak of the novel coronavirus (2019-nCoV), a new human-infecting β-coronavirus, has clearly demonstrated that the size of an organism does not reflect on its ability to affect an entire human population. 2019-nCOV, first detected in December 2019 in Wuhan, China, spread rapidly globally. Disease in humans ranged from flulike symptoms to severe acute hypoxic respiratory failure. The virus appears closely related to two bat-derived severe acute respiratory syndromes (SARS) coronaviruses. Although bats were likely the original host, animals sold at the Huanan seafood market in Wuhan might have been the intermediate host that enabled the emergence of the virus in humans. Under the electron microscope, the SARS-CoV-2 virus grips its receptor tighter than the virus behind the SARS outbreak in 2003 to 2004. The viral particle docks onto the angiotensin-converting enzyme 2 (ACE2) receptor and initiates viral entry. This review discusses the various aspects of the SARS-CoV-2 virus, its structure, pathophysiology, mechanism of interaction with human cells, virulence factors, and drugs involved in the treatment of the disease.
Keywords
antiviral treatment
coronavirus spike protein
cytokine storm
Introduction
A novel variety of coronavirus, the severe acute respiratory syndrome coronavirus 2 (SARS-CoV-2) virus, reported late in 2019 from Wuhan, central China, has now globally spread at a ruthless speed.1 It has expressed itself pandemically around our planet on all continents except Antarctica. The SARS-CoV-2 virus has been solely responsible and far exceeded the number of lives lost within a short period when compared with the 1918 pandemic and has had a significantly greater global economic impact.2 The World Health Organization (WHO) informed declared that the coronavirus pandemic is the “defining global health crisis of our time” and has revealed the best and worst in humanity. On March 22, 2020, Mr. Narendra Modi, the Prime Minister of India, highlighted the scale of the challenge as follows: “Even World War I and II didn't affect as many countries as the coronavirus has done.” SARS-CoV-2, the causative organism, is the name given to this virus; it is an enveloped ribosomal nucleic acid (RNA)-β coronavirus that among other manifestations causes the respiratory disease called coronavirus disease 2019 (COVID-19) (Fig. 1). Coronaviruses are a family of viruses, named for the crownlike effect created by spikes on their surface, which are surface proteins that help them invade human cells. Similar coronaviruses cause the common cold. What we are dealing with now is a new or novel coronavirus called SARS-CoV-2 that is effectively transmitted between humans and can cause a wide range of clinical conditions ranging from asymptomatic to a fatal infection in both adults and children.3

- Family of coronaviridae.
Structure of Coronavirus
Coronaviruses, a large family of viruses, are so called because of the crown-like appearance due to protein spikes on their surface that help the virus invade human cells. Coronaviruses belong to the Coronaviridae family in the order Nidovirales1, 4 and are classified into four groups: α, β, gamma, and delta. Alpha- and β-coronaviruses infect mammals, gamma-coronaviruses infect avian species, and delta-coronaviruses infect both mammalian and avian species (Fig. 1). The examples of the α-coronaviruses include human coronavirus NL63 (HCoV-NL63), porcine transmissible gastroenteritis coronavirus (TGEV), porcine epidemic diarrhea coronavirus (PEDV), and porcine respiratory coronavirus (PRCV). Beta-coronaviruses include SARS-CoV-1 and SARS-CoV-2, MERS-CoV (Middle East respiratory syndrome coronavirus), bat coronavirus HKU4, mouse hepatitis coronavirus (MHV), bovine coronavirus (BCoV), and human coronavirus OC43. Gamma- and delta-coronaviruses include avian infectious bronchitis coronavirus (IBV) and porcine delta-coronavirus (PdCV). Coronaviruses are remarkably large, enveloped, positive-stranded RNA viruses, and have the most voluminous genome, ranging from 27 to 32 kb.4 The nucleocapsid helical protein encloses the genome and is further surrounded by an envelope. The envelope has three structural proteins, namely, membrane (M) protein, envelope (E) protein, and spike protein (S) (Fig. 2a). The M and E proteins facilitate viral assembly, whereas the S protein is responsible for the viral attachment and entry into host cells. The S protein forms substantial protrusions from the virus surface, giving coronaviruses the look of having crowns (corona), is a key determinant of viral host response, and is a major facilitator of the host immune response.1
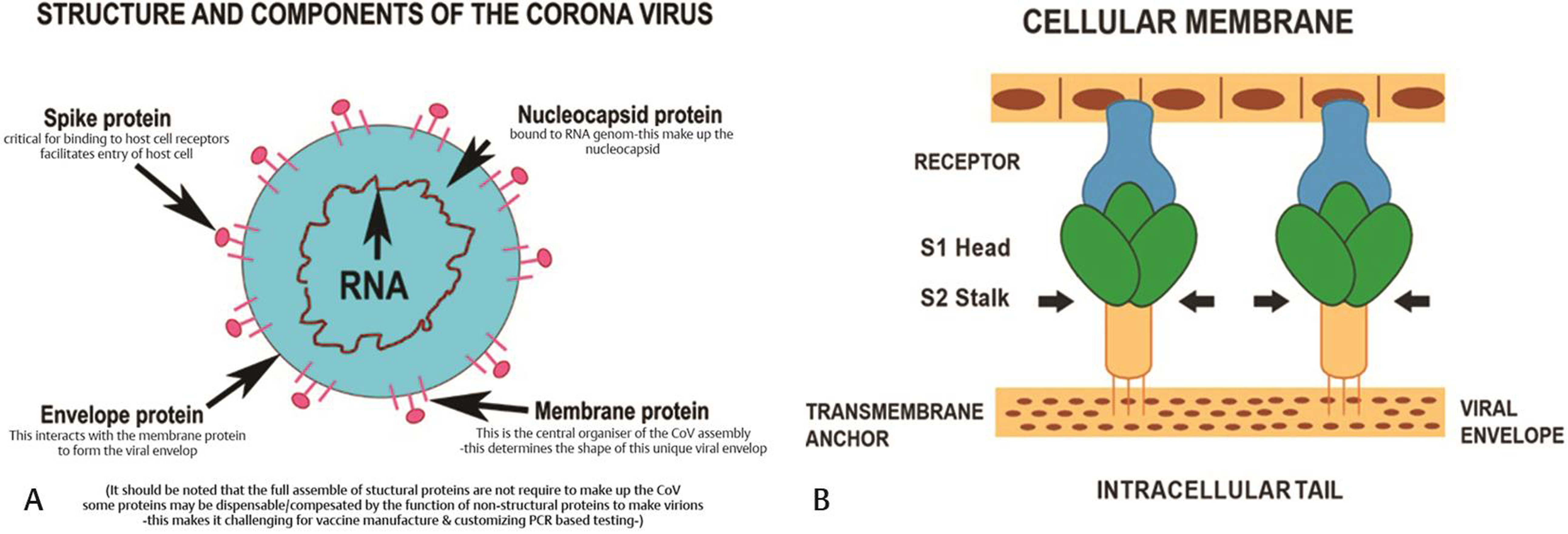
- (A) Structure and components of corona virus showing spike protein, envelop protein, membrane protein and nucleocapsid protein. (B) S protein is responsible for the viral attachment and entry into host cells.
The Life Cycle of SARS-COV-2
The invasion begins when the S protein binds to the host cellular angiotensin-converting enzyme 2 (ACE2) receptor (Fig. 3). Binding triggers a conformation change in the S protein that leads to viral envelope fusion with the host cell membrane through the endosomal pathway. SARS-CoV-2 virus then releases RNA into the host cell. Genome RNA is translated into viral replicase polyproteins pp1a and 1ab, which are then broken into smaller products by viral proteinases. The breakdown reaction produces a collection of subgenomic mitochondrial RNAs (mRNAs) by interrupted transcription and transformation into relevant viral proteins. Viral proteins and genomic RNA are collected into virions in the endoplasmic reticulum, and Golgi apparatus and then transported through vesicles that are released from the infected host cell. The SARS-CoV2 virus S protein has three segments: a large ectodomain, a single-pass transmembrane anchor, and a short intracellular tail. A receptor-binding subunit S1 and a membrane-fusion subunit S2 form the ectodomain. Electron microscopy studies indicate that the spike is like a clove-shaped trimer with three S1 heads and a trimeric S2 stalk.5, 6, 7, 8, 9, 6, 7, 8, 9, 10 When the virus enters the host cell, the S1 head binds to a receptor on the host cell surface, attaching the virus, enabling the S2 stalk to connect with the host and viral membranes, and allowing the viral genomes into the host cells (Fig. 2b). These crucial initial steps during coronavirus infection, namely receptor binding and membrane fusion, are primary targets for human therapeutic interventions.
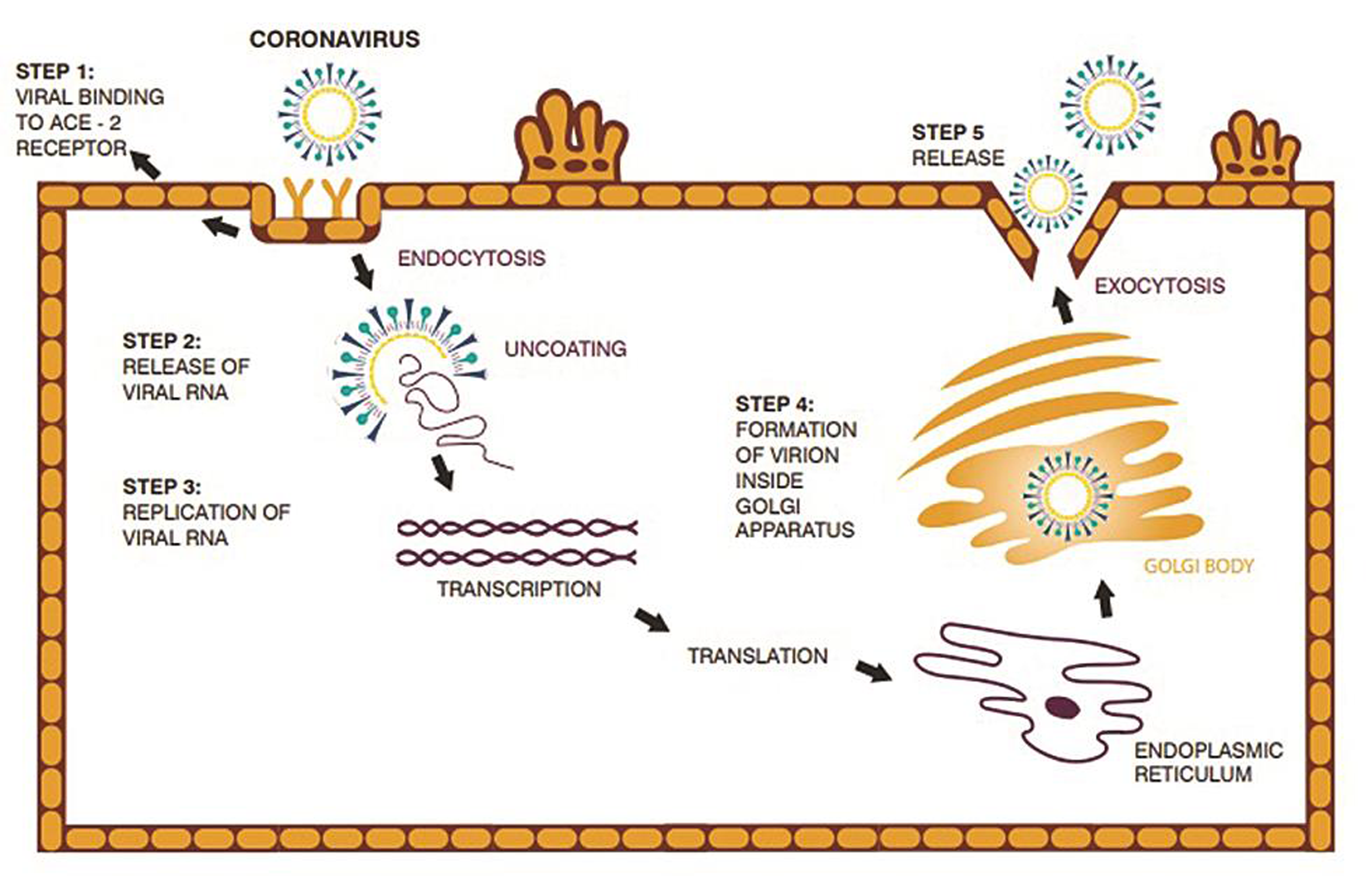
- The life-cycle of SARS-COV2 virus starts with invasion when the S protein binds to the host cellular angiotensin-converting enzyme 2 (ACE2) receptor.
Coronavirus and Receptor Binding
Coronaviruses demonstrate a pattern of receptor recognition.10 The α-coronavirus HCoV-NL63 and the β-corona SARS-CoV viruses, both recognize a zinc peptidase in the ACE2 receptor. The SARS-CoV-2 gains access into the cells by binding to the ACE2 receptor. Two binding hot spots have been identified on human ACE2: ACE2 residues Lys31 and Lys353.11, 12, 13, 14, 15, 16, 17, 18 These two hotspots contribute significantly to virus–receptor binding. The coronavirus exists in two distinct configurations: (1) prefusion trimeric spike containing three receptor-binding S1 heads and a trimeric membrane-fusion S2 stalk and (2) postfusion trimeric S2, which is a six-helix bundle with exposed fusion peptides. A variety of triggers regulate the transition of the spikes from the prefusion to the postfusion arrangements. Receptor attachment and membrane fusion are critical determinants of the host response, and tissue response is characteristic of coronavirus infection. As coronaviruses bind to the ACE2 receptor, it was proposed that ACE inhibitors (ACE-1) and angiotensin receptor blockers (ARBs) may be associated with increased severity of illness among COVID-19 patients due to ACE2 receptor upregulation.19, 20 Researchers have also hypothesized that inhibition of the renin–angiotensin system and increased levels of ACE2 may have a protective effect in acute lung injury. In a recent study evaluating a cohort of 205 hospitalized patients with COVID-19 infection, although ACE-1 did not affect the severity of illness, it did provide a beneficial effect. In this study, 37 (18%) were on ACE-1, and a serial logistic regression analysis confirmed a significant decrease in the primary end points of death or admission to the intensive care unit (ICU) in those on ACE-I compared with those not on ACE-I.21, 22 The possible protective and treatment effectiveness of ARBs (losartan) is currently being evaluated in paired multicenter randomized double-blind controlled clinical trials initiated at the University of Minnesota (ClinicalTrials.gov NCT04311177 and NCT04312009).
The Cytokine Storm and Secondary Hemophagocytic Histiocytosis
A devastating, rapidly fatal cytokine storm may occur in coronavirus infections resulting in secondary hemophagocytic lymphohistiocytosis (sHLH) characterized by multiorgan failure (Fig. 4). This cytokine storm is responsible for much of the problems encountered by patients with the SARS-CoV-2 infection because the storm is a hyperimmune response to the virus, resulting in a dysregulated and accelerated expression of proinflammatory cytokines interleukin (IL)-2, IL-6, IL-8, ILs, and tumor necrosis factor (TNF). The predominant feature of sHLH includes reduced white blood cell counts, specifically lymphopenia, elevated serum ferritin levels, and severe acute respiratory distress syndrome (ARDS). High ferritin levels are particularly dangerous because they suggest the presence of a significant hyperinflammatory response with their consequences on the lung.23, 24 Thus, the treatment goals are to lower the consequences of the severe cytokine storm during severe COVID-19 pneumonia. Since SARS-CoV-2 entry in cells is dependent on the connection of viral proteins S with cellular receptors and activation of viral proteins by proteases of host cells, this could be one area of virus inhibition. Thus, factors that affect the clathrin-mediated endocytosis (a procedure that is in part regulated by microtubules remodelling) could potentially decelerate viral infection of cells.25 Colchicine is another drug that could be helpful because it has high bioavailability in granulocytes and monocytes. Its property to bind unpolymerized tubulin heterodimers to form a stable complex effectively inhibits microtubule dynamics and is a nonselective inhibitor of the NLRP3 inflammasome, a major pathway element in the development of ARDS.26 The options for cytokine inhibition include possible corticosteroid or intravenous immunoglobulin administration. A randomized controlled trial with tocilizumab, an IL-6 blocker, is currently recruiting patients in China.27 Other treatment options are based on clinical findings and include heparin, serine protease inhibitors such as ulinastatin, high-dose vitamin C, continuous renal replacement therapy (CRRT), and high-volume hemofiltration as adjuncts in the care of critically ill patients with COVID-19 infection. Anti-IL-1 therapy with anakinra is being evaluated for patients with severe COVID-19 and sHLH.28 Evidence for a severe cytokine storm and mortality includes reports by Tu et al, reporting higher levels of IL-6, C-reactive protein (CRP), and D-dimer levels in nonsurvivors.29, 30 These findings offer information regarding the characteristics of severe COVID-19 infection and support further investigation regarding the use of immunomodulators.31 An expert consensus from China recommended cytokine clearance using an artificial liver blood purification systems. Plasma exchange, plasma absorption, and hemofiltration or plasma filtration have also been considered as alternative therapies.32, 33
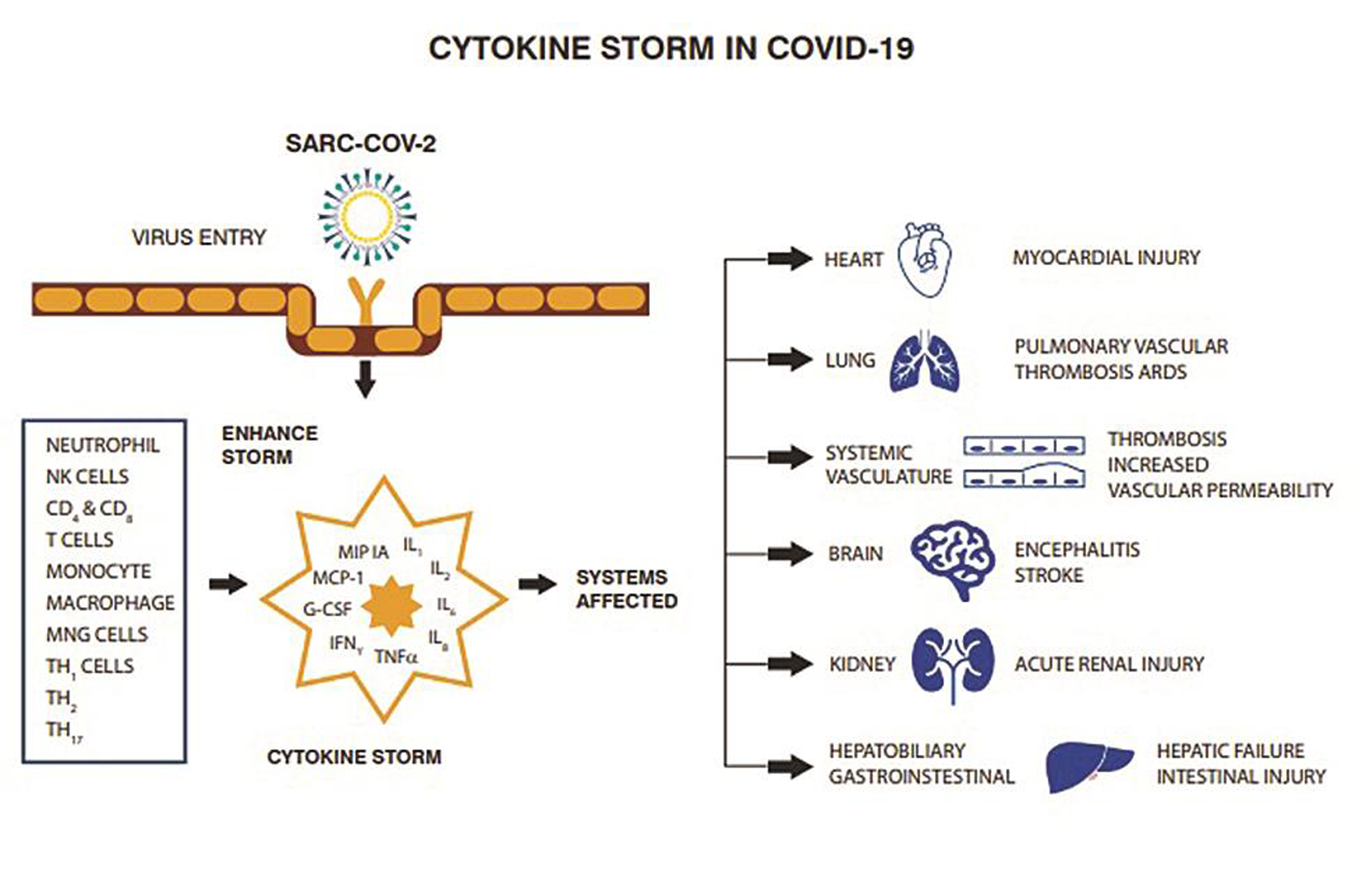
- Cytokine storm may occur in infection with coronavirus characterized by multiorgan failure.
Coronavirus and Hemoglobin Metabolism
The pathological mechanism of the novel coronavirus causing COVID-19 remains enigmatic and mysterious.34 A report that looked at biochemical indices of 99 patients with novel coronavirus pneumonia demonstrated abnormal hemoglobin metabolism.35 This article revealed a decrease in the hemoglobin and neutrophil counts associated with elevated levels of serum ferritin, erythrocyte sedimentation rate, CRP, albumin, and lactate dehydrogenase.
Exclusive Molecular Mechanism Explaining COVID-19 Expression33
COVID-19 may not resemble the usual types of ARDS as seen in our routine critical care practice. The key pathogenic molecular step of SARS-Cov-2 is to attack the 1-β chain of hemoglobin, attacking the porphyrins, dissociating, and releasing iron into the circulation.36 The ORF1ab, ORF10, and ORF3a proteins of the virus attack the heme on the 1-β chain of hemoglobin.37 The virus binds deoxygenated hemoglobin readily compared with oxygenated hemoglobin, resulting in resistant hypoxia coupled with rapid multiorgan failures. Following hemolysis of red blood cells, viral proteins bind to the hemoglobin and the virus enters the host cell through the spike-CD147 pathway. The virus interferes with the heme anabolic pathway and causes the disease. The free iron released into the circulation is toxic, causing powerful oxidative damage to the lungs. Free iron toxicity results in inflammation of alveolar macrophages, which leads to characteristic changes seen on computed tomography (CT) scans of the lungs.38 The host attempts to compensate by accelerating hemoglobin synthesis consistent with improving hemoglobin values noted in these patients.39 Another compensatory mechanism that addresses the iron load is the increased ferritin levels documented in these patients.40 One cause for monocytosis seen in these patients may be the need for additional macrophages to engulf the excess iron load. The cause of lymphopenia may be that during white blood cell differentiation, the monocytes line is favored rather than lymphocytes line. As the iron load and hemoglobin increase, blood viscosity also increases. This in combination with the hypercoagulable state may cause diffuse micro- and macrocirculatory thrombosis and the markedly elevated D-dimer levels seen in these patients.41 Postmortem studies have demonstrated that the ARDS picture is misleading and that the primary pathologic process is disseminated thrombosis. Mechanical ventilation without addressing this issue and may cause more lung damage. Early and aggressive anticoagulation can be lifesaving in these patients.42 Chloroquine (CQ) phosphate competes with porphyrin and binds to the viral protein, thereby inhibiting the viral protein's attacking the heme and the binding to porphyrin.
Thrombogenesis and Coagulopathy in COVID-19
A fulminant coagulopathy is described in patients with COVID-19 pneumonia. The hypercoagulable state is created by endothelial dysfunction, leading to excessive thrombogenesis and inhibition of fibrinolysis. Hypoxia is a noted trigger of the procoagulant pathway, leading to venous thrombosis. Postmortem examination of patients who died following a critical illness due to COVID-19 demonstrated micro thrombosis in the pulmonary vessels.43, 44 In a study of 183 consecutive patients with COVID-19, D-dimer and fibrin degradation products were significantly elevated, and the prothrombin and partial thromboplastin times were higher among nonsurvivors as compared with survivors. Among nonsurvivors, 71.4% had evidence of disseminated intravascular coagulation compared with 0.6% among survivors.45 The incidence of thrombotic events, including acute pulmonary embolism, deep vein thrombosis, acute ischemic stroke, acute myocardial infarction, and arterial embolism, was evaluated in 182 COVID-19 patients admitted to three hospitals in the Netherlands. Thrombotic events were noted in 31% of patients in this study, with acute pulmonary embolism being the most common complication (82%). Increasing age and the presence of coagulopathy were independent predictors of thrombotic events.46 Clinically significant coagulopathy with the presence of antiphospholipid antibodies was reported among three patients with COVID-19.44, 47 In a retrospective, observational study COVID-19 patients who received anticoagulant therapy with unfractionated or low-molecular-weight heparin were contrasted with those who had no anticoagulant treatment. A sepsis-induced coagulopathy score (SIC) was calculated based on platelet count, international normalized ratio (INR), and the sequential organ failure (SOFA) score. On multivariate logistic regression analysis, patients with an SIC score of ≥4 who were treated with unfractionated or low-molecular-weight heparin had a significantly lower 28-day mortality compared with those who did not receive anticoagulant therapy.48 Thus, anticoagulation should be part of the therapy of patients with COVID-19. In view of a high incidence of thrombotic complications among patients with COVID-19, the International Society of Thrombosis and Haemostasis recommended the administration of prophylactic low-molecular-weight heparin to all hospitalized patients with COVID-19 in the absence of active bleeding, ensuring that platelet counts are greater than 25,000/μL regardless of the INR and activated partial thromboplastin time. This strategy is expected to reduce the incidence of a sepsislike coagulopathy and prevents venous thromboembolism.49
Drugs Used for COVID-19 Treatment
According to the WHO, there are neither available vaccines nor specific antiviral treatments for COVID-19. Care for patients with COVID-19 includes isolation, social distancing, hygiene, treatment of symptoms, supportive care, and institution of experimental protocols. On 1 May 2020, the United States gave Emergency Use Authorization to the antiviral remdesivir for people hospitalized with severe COVID-19.31 In March, WHO initiated the “SOLIDARITY Trial” to assess the treatment efficacy of four existing antiviral compounds, which are favipiravir, remdesivir, lopinavir, and hydroxychloroquine ([HCQ] or CQ).50 On March 16, 2020, the first clinical trial of a vaccine started, which consists of a harmless genetic code copied from the virus that causes the disease in Seattle, United States.42 The following are the drugs suggested from the literature searches against coronavirus disease (Fig. 5 Table 1).51
Drugs |
Mechanism of action |
Status of the clinical drug trial |
---|---|---|
Abbreviations: ACE2, angiotensin-converting enzyme 2; COVID-19, coronavirus disease 2019; CSF2, colony-stimulating factor 2; DIC, disseminated intravascular coagulation; GM-CSF, granulocyte-macrophage colony-stimulating factor; HCQ, hydroxychloroquine; IFN, interferon; IL, interleukin; IL-1RA, IL-1 receptor antagonist; iNO, inhaled nitric oxide; IVIG, intravenous gamma globulin; LHQW, Lianhua Qingwen; mAb, monoclonal antibody; MERS-CoV, Middle East respiratory syndrome coronavirus; NK, natural killer; NSAIDs, nonsteroidal anti-inflammatory drugs; PBMC, protein peripheral blood mononuclear cell; rhACE2, recombinant human angiotensin-converting enzyme 2; RNA, ribosomal nucleic acid; S, spike; SARS-CoV-2, severe acute respiratory syndrome coronavirus 2; TCM, traditional Chinese medicine; TMPRSS2, transmembrane protease/serine subfamily member 2; VEGF, vascular endothelial growth factor. |
||
Antiviral |
||
Remdesivir |
Antiviral interferes with virus RNA polymerases to inhibit virus replication |
U.S. Food and Drug Administration permitted emergency use authorization on May 1, 2020; clinical trials have been initiated in India |
Favipiravir |
Antiviral inhibits viral RNA polymerase, thus interfering with viral replication |
Two clinical trials have achieved their primary end points |
Lopinavir/ritonavir |
Antiviral protease inhibitors |
Trials did not achieve theirprimary end points |
Oseltamivir (Tamiflu) |
Antiviral drug approved for the treatment of influenza A and B; it targets the neuraminidase distributed on the surface of the influenza virus to inhibit the spread of the virus |
Under evaluation |
Umifenovir (Arbidol) |
Antiviral impedes trimerization of SARS-CoV-2 spike glycoprotein and inhibits host cell adhesion like that of influenza virus hemagglutinin |
Under evaluation |
EIDD-2801 |
Antiviral incorporated during RNA synthesis and then drives mutagenesis, thus inhibiting viral replication |
Prepared for trial |
CD24Fc |
Antiviral immunomodulator against inflammatory response |
Under evaluation |
Antimalarial |
||
Chloroquine/hydroxychloroquine |
Antimalarial endosomal acidification fusion inhibitor anti-inflammatory activity |
Reduction of COVID-19 virus load reported; results from ongoing clinical trials awaited; its recommendation for treatment has been withdrawn by multiple agencies due to potential toxicity; does not help postexposure51 |
Anticoagulants |
||
Heparin |
Anticoagulants reverse the hypercoagulability in severe cases |
Proven Trial |
Anti-inflammatory |
||
Ibuprofen |
NSAIDs Anti-inflammatory |
Controversial: avoid if usual contraindications present |
Colchicine |
Anti-inflammatory used in gout; inhibitory effects on macrophages; in COVID-19 with cardiomyopathy, it has been shown to reduce inflammation in the cardiac myocytes |
Under evaluation |
Anakinra |
Modified human IL-1RA used in rheumatoid arthritis |
Under evaluation |
Immunological |
||
Tocilizumab and sarilumab |
Humanized mAb targeting IL-6 |
Under evaluation |
Bevacizumab |
Humanized mAb targeting VEGF |
Under evaluation |
Baricitinib |
Attenuates proinflammatory response by inhibiting JAK and blocks virus entering host cells through inhibiting AAK1 |
Under evaluation |
Lenzilumab |
Humanized monoclonal antibody that targets CSF2/GM-CSF |
Under evaluation |
IFNs |
Immune enhancer inhibits viral RNA transcription, protein translation, and posttranslational modification, thus suppress virus replication |
Under evaluation |
NK cell therapy |
Immune enhancer direct cytotoxicity and immunomodulatory capability |
Under evaluation |
IVIG |
Immune enhancer passive immunity and anti-inflammatory effects |
Under evaluation |
Corticosteroids |
Reduces proinflammatory cytokines and possess antifibrotic properties |
Low dose recommended |
Cepharanthine/selamectin/mefloquine hydrochloride |
Inhibit infection of simian Vero E6 cells with pangolin coronavirus, whose S protein shares 92.2% amino acid identity with that of SARS-CoV-2; prevents viral entry |
Under evaluation |
Antihelminthic |
||
Niclosamide and Ivermectin |
Anthelmintic drug Virus replication inhibitor |
Under evaluation |
Nitazoxanide and Tizoxanide |
Suppress proinflammatory cytokines in PBMCs and IL-6 in vivo |
Under evaluation |
Alternative medicine |
||
Chinese medicines |
||
LHQW |
TCM prevention and treatment for influenzas |
Under evaluation |
Xuebijing injection |
TCM endotoxin antagonist, anti-inflammatory agent and anti-coagulant is used for sepsis |
Under evaluation |
Antibacterial |
||
Azithromycin |
Antibacterial proven to be active in vitro against Zika and Ebola viruses |
Positive data for its use, along with hydroxychloroquine, in a COVID-19 clinical trial; not recommended in combination with HCQ because of cardiac arrhythmias |
Miscellaneous |
||
Inhibitors of TMPRSS2 serine protease |
Cleavage and activation of the S protein of SARS-CoV that is required for membrane fusion and host cell entry is mediated by TMPRSS2 |
Under evaluation |
rhACE2 |
ACE2 blocker binds to virus S protein, thus protects host lungs from virus attack |
Under evaluation |
iNO |
Vasodilator potent and selective pulmonary vasodilation and antimicrobial activity |
Under evaluation |
Camostat mesilate (Foipan |
Synthetic serine protease inhibitors were developed for the treatment of oral squamous cell carcinoma, dystrophic epidermolysis, exocrine pancreatic enzyme inhibition, and chronic pancreatitis |
Under evaluation |
Nafamostat mesilate (Buipel) |
A synthetic serine protease inhibitor approved in Japan for the treatment of acute pancreatitis, DIC, and anticoagulation in extracorporeal circulation; it inhibits MERS-CoV S protein-mediated viral membrane fusion with TMPRSS2-expressing lung Calu-3 host cells by inhibiting TMPRSS2 protease activity |
Under evaluation |
Dapagliflozin |
Used to treat type 2 diabetes and, with certain restrictions, type 1 diabetes, and adults with heart failure with reduced ejection fraction to reduce the risk of cardiovascular death |
Under evaluation |
Convalescent plasma |
Antiviral plasma from recovered patients provides protective antibody |
Early trials showing promising results |
Supportive |
||
Vitamin C |
Boosts immunity by stimulating IFN production, supplying lymphocyte proliferation, and enhancing neutrophil phagocytic capability |
Under evaluation |
Vitamin D |
Induces secretion of antimicrobial peptides and has immunomodulatory property |
Under evaluation |
Zinc |
necessary for the immune system and has antiviral activities |
Under evaluation |
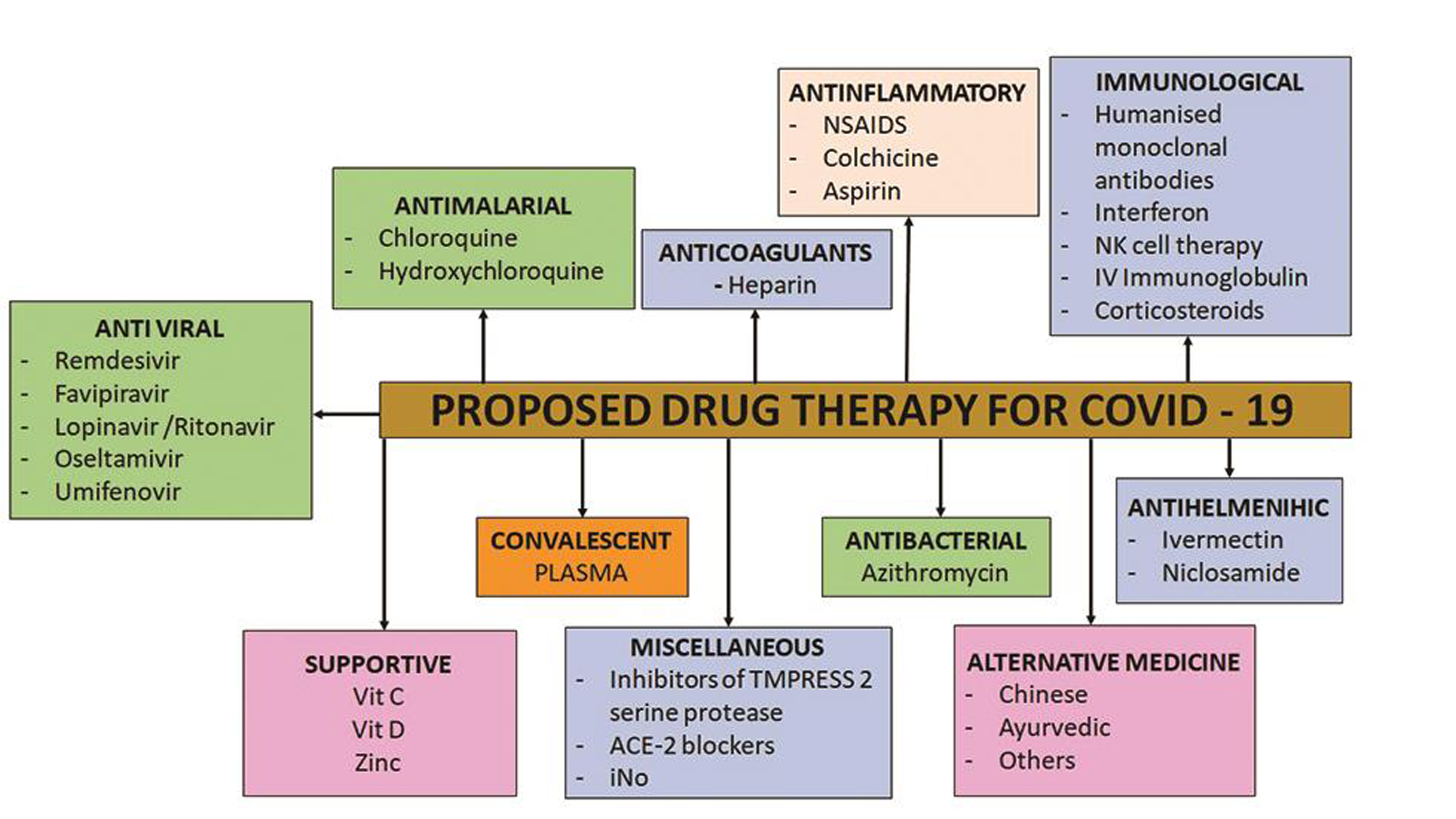
- Pharmacological approach to the proposed treatment of Covid-19 infection”
Hydroxychloroquine
There is contradictory evidence regarding the use of HCQ in COVID-19 infection. CQ, which is used in treating malarial and autoimmune diseases, also confers considerable broad-spectrum antiviral effects even against SARS-CoV. HCQ is chemically like CQ but with lower ocular toxicity and has proven to be efficacious in containing SARS-CoV-2 in vitro.52, 53 CQ phosphate inhibits terminal phosphorylation of ACE2, and HCQ elevates the pH in endosomes, which participate in virus cell entry. While HCQ may have benefit, it appears that additional investigation is required before committing to the routine use of HCQ against COVID-19.54
QT Prolongation with the Hydroxychloroquine–Azithromycin Combination
Both HCQ and azithromycin are known to prolong the QT interval. Thus, patients on this combination require close cardiac monitoring. The combination has been used successfully in some reports,55 with in vitro efficacy of the combination.56 Chorin et al describe the occurrence of significant QT prolongation including the occurrence of Torsade de Pointes when such a combination is used in the treatment of COVID-19.57 Thus, regular monitoring is required, especially among patients with renal dysfunction.
Remdesivir
The nucleoside analogue remdesivir has in vitro activity against SARS-CoV-2.58 It was originally developed to treat the Ebola virus disease. It is an adenosine analogue, which inserts into viral RNA chains, causing the premature breaking of the chains.58 On May 1, 2020, the U.S. Food and Drug Administration granted Gilead Sciences Inc. Emergency Use Authorization of remdesivir to be prescribed by licensed health care providers to treat adults and children hospitalized with severe COVID-19.59 Severe COVID-19 is defined as patients with an oxygen saturation (SpO2) of ≤94% on room air or requiring supplemental oxygen, mechanical ventilation, or extracorporeal membrane oxygenation (ECMO), a heart–lung bypass machine. It was administered on a compassionate basis to 61 patients with COVID-19 who had an oxygen saturation of less than 94% on room air or required supplemental oxygen. Remdesivir was administered intravenously in a dose of 200 mg on day 1 followed by 100 mg per day for 9 days. Clinical outcomes of 53 of the 61 patients were analyzed. At baseline, 30 (57%) patients were invasively ventilated and 4 were on ECMO. The median follow-up period was 18 days. The level of the oxygen support device (ECMO, invasive mechanical ventilation, noninvasive ventilation, high- or low-flow oxygen) could be successfully weaned in 36 (68%) patients. Of the 30 patients, 17 (57%) who were invasively ventilated were successfully liberated from the ventilator extubated. Twenty-five (47%) patients had been discharged, and seven (13%) had died at the time of follow-up. The mortality was 18% (6/34) among invasively ventilated patients and 5% (1/19) among those who did not receive invasive ventilation.60
Favipiravir
Favipiravir is an antiviral used against influenza.61 It is an oral pyrazine carboxamide derivative and guanine analogue developed by Toyama Chemical, Tokyo, Japan. Favipiravir selectively inhibits the RNA-dependent RNA polymerase of RNA viruses and induces lethal RNA transversion mutations, thereby producing a nonviable virus phenotype; this phenotype cannot bind to E2 glycoprotein and nucleocapsid, and its binding energy to viral E protein, ORF7a, and ORF1ab is higher than it is to porphyrin. The binding energy of E protein and favipiravir is more than 2,700 times the binding energy of porphyrin. The primary function of E protein is to help the virus enter host cells, which suggests that favipiravir acts by effectively preventing the viral entrance to human host cells. The WHO and the European Union has initiated clinical trials to test remdesivir, CQ, and HCQ, lopinavir/ritonavir (LPV-R), and LPV-R plus interferon (INF) β-1a in COVID-19 patients worldwide in the SOLIDARITY Trial and in the DisCoVeRy Trial.62, 63, 64
Lopinavir/Ritonavir with or without Interferon β-1A
LPV-R is a specific protease inhibitor, fixed-dose combination medication used for the treatment and prevention of human immunodeficiency virus (HIV). INF β-1a is a cytokine in the INF family used to treat multiple sclerosis (MS) produced by mammalian cells. Concomitant use of ritonavir and lopinavir could increase the plasma half-life of lopinavir through cytochrome P450 inhibition in the liver. Kim et al evaluated triple combination therapy with LPV-R, ribavirin, and IFN and showed clinical effectiveness for MERS.65 A randomized controlled trial (MIRACLE Trial) was initiated to determine the therapeutic efficacy of LPV-R combined with INF β-1b in patients infected with MERS-CoV.66 Studies observed that treatment with LPV-R compared with the standard care group was not associated with any change in time to clinical improvement and that mortality at 28 days was similar in both groups. Treatment with LPV-R did not reduce viral RNA load and SARS-CoV-2 RNA remained detectable at 28 days in 40.7% of the patients in the LPV-R cohort. However, patients in the LPV-R cohort demonstrated fewer complications, had lesser need for invasive respiratory support, and had fewer secondary infections than did patients who did not receive LPV-R treatment.67
Passive Antibodies
The transfusion of convalescent plasma collected from patients who had recovered from COVID-19 to patients who were newly infected is being investigated in an active clinical trial initiated at Mayo Clinic (Expanded Access to Convalescent Plasma for the Treatment of Patients with COVID-19; ClinicalTrials.gov Identifier: NCT04338360). Transferring purified and concentrated antibodies through transfusion of convalescent plasma is a method of passive immunization. Neutralization of the virus is expected with this therapy, and antibody-dependent cellular cytotoxicity and subsequent phagocytosis may be possible.68
Steroids
Steroid administration has shown to benefit patients in the acute phase of the disease.69 The WHO does not currently recommend corticosteroid therapy in other viral diseases such as for patients with dengue. One concern is that the glucocorticoid-mediated stimulation of the hypothalamic–pituitary–adrenal axis may drive lymphocytopenia or promote exaggerated proinflammatory responses, which eventually worsen the pathogenic condition.69, 70 According to the surviving sepsis guidelines, there was a reduced length of stay in the ICU and, in turn, reduced cost with the use of low-dose steroids.71, 72
Vaccine Trials
Several phase 1 vaccine trials are already underway.73, 74, 75, 76, 77, 78, 79
Conclusion
COVID-19 has been the infection of the century and has surprised clinicians and scientists with its structure and mechanism of infecting host cells, mimicking ARDS, and enveloping hemoglobin, resulting in severe hypoxia with an intense immune response and multiorgan failure. The molecular docking technology identified the binding site of viral proteins to porphyrin. The virus infects cells with ACE2 receptors and the immune cells produce antibodies, leading to immune-mediated hemolysis. The elevated levels of inflammation result in a cytokine storm, causing multiple organ failure. Lung damage occurs with thrombosis and leads to hypoxia. Various drug trials are ongoing to find the ultimate cure for this viral disease. Careful selection should be made from the extensive list of drugs available, with adequate knowledge about their side effects, and, most importantly, prevention being the ultimate mantra in the treatment of COVID-19.
Acknowledgment
The authors would like to thank Mr Pavan Krishna Kanchi, MS, Chemical Engineering, PhD Scholar, Indian Institute of Technology, Guwahati, India, for helping in making the first draft of the manuscript, and Mr Sigesh AK, Department of Marketing and Development, Narayana Health, Bangalore, for helping us with the editing of diagrams.
Conflict of Interest
None declared.
Sources of Support None.
References
- DownToEarth. Between 1918–19 Spanish flu and COVID-19, not much has changed. https://www.downtoearth.org.in/blog/governance/between-1918-19-spanish-flu-and-covid-19-not-much-has-changed-71425
- Liang W, Liang H, Ou L, et al; China Medical Treatment Expert Group for COVID-19. Development and validation of a clinical risk score to predict the occurrence of critical illness in hospitalized patients with COVID-19. JAMA Intern Med 2020
- Full-genome sequences of the first two SARS-CoV-2 viruses from India. Indian J Med Res. 2020;151:200-209. (2 & 3)
- [Google Scholar]
- Pre-fusion structure of a human coronavirus spike protein. Nature. 2016;531:118-121. (7592)
- [Google Scholar]
- Cryo-electron microscopy structure of a coronavirus spike glycoprotein trimer. Nature. 2016;531:114-117. (7592)
- [Google Scholar]
- Architecture of the SARS coronavirus prefusion spike. Nat Struct Mol Biol. 2006;13(8):751-752.
- [Google Scholar]
- Conformational states of the severe acute respiratory syndrome coronavirus spike protein ectodomain. J Virol. 2006;80(14):6794-6800.
- [Google Scholar]
- Structural basis for the recognition of SARS-CoV-2 by full-length human ACE2. Science. 2020;367:1444-1448. (6485)
- [Google Scholar]
- Receptor recognition mechanisms of coronaviruses: a decade of structural studies. J Virol. 2015;89(4):1954-1964.
- [Google Scholar]
- Structural analysis of major species barriers between humans and palm civets for severe acute respiratory syndrome coronavirus infections. J Virol. 2008;82(14):6984-6991.
- [Google Scholar]
- A virus-binding hot spot on human angiotensin-converting enzyme 2 is critical for binding of two different coronaviruses. J Virol. 2011;85(11):5331-5337.
- [Google Scholar]
- Mechanisms of host receptor adaptation by severe acute respiratory syndrome coronavirus. J Biol Chem. 2012;287(12):8904-8911.
- [Google Scholar]
- Novel antibody epitopes dominate the antigenicity of spike glycoprotein in SARS-CoV-2 compared to SARS-CoV. Cell Mol Immunol. 2020;17(5):536-538.
- [Google Scholar]
- Structure of the SARS-CoV-2 spike receptor-binding domain bound to the ACE2 receptor. Nature. 2020;581:215-220. (7807)
- [Google Scholar]
- Structural and functional basis of SARS-CoV-2 entry by using human ACE2. Cell. 2020;181(4):894-904.e9.
- [Google Scholar]
- Structural genomics of SARS-CoV-2 indicates evolutionary conserved functional regions of viral proteins. Viruses. 2020;12(4):E360.
- [Google Scholar]
- The SARS-CoV-2 exerts a distinctive strategy for interacting with the ACE2 human receptor. Viruses. 2020;12(5):E497.
- [Google Scholar]
- Reply to the Comment by Dr. Cure on “Should COVID-19 concern nephrologists? Why and to what extent? The emerging impasse of angiotensin blockade.”. Nephron. 2020;144(5):253-254.
- [Google Scholar]
- Perico L, Benigni A, Remuzzi G. Should COVID-19 concern nephrologists? Why and to what extent? The emerging impasse of angiotensin blockade. Nephron 2020
- Angiotensinconverting-enzyme inhibitors (ACE inhibitors) and angiotensin II receptor blocker (ARB) use in COVID-19 prevention or treatment: a paradox. Infect Control Hosp Epidemiol 2020 (e-pub ahead of print)
- [CrossRef] [Google Scholar]
- Treatment with ACE-inhibitors is associated with less severe SARS-Covid-19 infection in a multi-site UK acute Hospital Trust. medRxiv 2020 (e-pub ahead of print)
- [CrossRef] [Google Scholar]
- Ruan Q, Yang K, Wang W, Jiang L, Song J. Clinical predictors of mortality due to COVID-19 based on an analysis of data of 150 patients from Wuhan, China. Intensive Care Med 2020
- Anti-spike IgG causes severe acute lung injury by skewing macrophage responses during acute SARS-CoV infection. JCI Insight. 2019;4(4):e123158.
- [Google Scholar]
- COVID-19: combining antiviral and anti-inflammatory treatments. Lancet Infect Dis. 2020;20(4):400-402.
- [Google Scholar]
- Colchicine: a potential therapeutic tool against COVID-19. Experience of 5 patients [in Spanish] Reumatol Clin 2020 (e-pub ahead of print)
- [CrossRef] [Google Scholar]
- Chinese Clinical Trial Registry. Available at: http://www.chictr.org.cn/searchprojen.aspx.
- Favorable anakinra responses in severe COVID-19 patients with secondary hemophagocytic lymphohistiocytosis. Cell Host Microbe. 2020;28(1):117-123.e1.
- [Google Scholar]
- Current epidemiological and clinical features of COVID-19; a global perspective from China. J Infect. 2020;81(1):1-9.
- [Google Scholar]
- Clinicolaboratory study of 25 fatal cases of COVID-19 in Wuhan. Intensive Care Med. 2020;46(6):1117-1120.
- [Google Scholar]
- LinksMedicus - Free Medical News & Perspectives. COVID-19 Updates. Available at: https://www.linksmedicus.com/news/coronavirus-disease-covid-19-updates/.
- A promising anti-cytokine-storm targeted therapy for COVID-19: the artificial-liver blood-purification. Syst Eng 2020 (e-pub ahead of print)
- [CrossRef] [Google Scholar]
- Potential effect of blood purification therapy in reducing cytokine storm as a late complication of critically ill COVID-19. Clin Immunol. 2020;214:108408.
- [Google Scholar]
- Transmission of 2019-nCoV Infection from an Asymptomatic Contact in Germany. N Engl J Med. 2020;382(10):970-971.
- [Google Scholar]
- Epidemiological and clinical characteristics of 99 cases of 2019 novel coronavirus pneumonia in Wuhan, China: a descriptive study. Lancet. 2020;395:507-513. (10223)
- [Google Scholar]
- COVID-19 disease: ORF8 and surface glycoprotein inhibit heme metabolism by binding to porphyrin. Available at: https://www.researchgate.net/publication/339748594_COVID-19_Disease_ORF8_and_Surface_Glycoprotein_Inhibit_Heme_Metabolism_by_Binding_to_Porphyrin.
- Wenzhong L, Hualan L. COVID-19: attacks the 1-beta chain of hemoglobin and captures the porphyrin to inhibit human heme metabolism. https://chemrxiv.org/articles/COVID-19_Disease_ORF8_and_Surface_Glycoprotein_Inhibit_Heme_Metabolism_by_Binding_to_Porphyrin/11938173
- Clinical features of COVID-19 in elderly patients: a comparison with young and middle-aged patients. J Infect. 2020;80(6):e14-e18.
- [Google Scholar]
- Dynamic changes in routine blood parameters of a severe COVID-19 case. Clin Chim Acta. 2020;508:98-102.
- [Google Scholar]
- Hematological findings and complications of COVID-19. Am J Hematol. 2020;95(7):834-847.
- [Google Scholar]
- COVID-19-related severe hypercoagulability in patients admitted to intensive care unit for acute respiratory failure. Thromb Haemost. 2020;120(6):998-1000.
- [Google Scholar]
- COVID-19 and its implications for thrombosis and anticoagulation. Blood. 2020;135(23):2033-2040.
- [Google Scholar]
- Autopsy findings and venous thromboembolism in patients with COVID-19: a prospective cohort study. Ann Intern Med 2020 (e-pub ahead of print)
- [CrossRef] [Google Scholar]
- Pulmonary vascular endothelialitis, thrombosis, and angiogenesis in Covid-19. N Engl J Med. 2020;383(2):120-128.
- [Google Scholar]
- Abnormal coagulation parameters are associated with poor prognosis in patients with novel coronavirus pneumonia. J Thromb Haemost. 2020;18(4):844-847.
- [Google Scholar]
- Confirmation of the high cumulative incidence of thrombotic complications in critically ill ICU patients with COVID-19: an updated analysis. Thromb Res. 2020;191:148-150.
- [Google Scholar]
- Coagulopathy and antiphospholipid antibodies in patients with Covid-19. N Engl J Med. 2020;382(17):e38.
- [Google Scholar]
- Anticoagulant treatment is associated with decreased mortality in severe coronavirus disease 2019 patients with coagulopathy. J Thromb Haemost. 2020;18(5):1094-1099.
- [Google Scholar]
- ISTH interim guidance on recognition and management of coagulopathy in COVID-19. J Thromb Haemost. 2020;18(5):1023-1026.
- [Google Scholar]
- Kupferschmidt K. WHO launches global megatrial of the four most promising coronavirus treatments. Science 2020
- A randomized trial of hydroxychloroquine as postexposure prophylaxis for Covid-19. N Engl J Med 2020 (e-pub ahead of print)
- [CrossRef] [Google Scholar]
- Pharmacokinetics of hydroxychloroquine and its clinical implications in chemoprophylaxis against malaria caused by plasmodium vivax. Antimicrob Agents Chemother. 2009;53(4):1468-1475.
- [Google Scholar]
- Hydroxychloroquine, a less toxic derivative of chloroquine, is effective in inhibiting SARS-CoV-2 infection in vitro. Cell Discov. 2020;6(1):16.
- [Google Scholar]
- Review: hydroxychloroquine and chloroquine for treatment of SARS-CoV-2 (COVID-19) Open Forum Infect Dis. 2020;7(4):a130.
- [Google Scholar]
- Full-length title: early treatment of COVID-19 patients with hydroxychloroquine and azithromycin: a retrospective analysis of 1061 cases in Marseille, France. Travel Med Infect Dis 2020 (e-pub ahead of print)
- [CrossRef] [Google Scholar]
- In vitro testing of combined hydroxychloroquine and azithromycin on SARS-CoV-2 shows synergistic effect. Microb Pathog. 2020;145:104228.
- [Google Scholar]
- QT interval prolongation and torsade de pointes in patients with COVID-19 treated with hydroxychloroquine/azithromycin. Heart Rhythm 2020 (e-pub ahead of print)
- [CrossRef] [Google Scholar]
- The antiviral compound remdesivir potently inhibits RNA-dependent RNA polymerase from Middle East respiratory syndrome coronavirus. J Biol Chem. 2020;295(15):4773-4779.
- [Google Scholar]
- Remdesivir for the treatment of Covid-19 - preliminary report. N Engl J Med 2020 (e-pub ahead of print)
- [CrossRef] [Google Scholar]
- Compassionate use of remdesivir for patients with severe Covid-19. N Engl J Med. 2020;382(24):2327-2336.
- [Google Scholar]
- Favipiravir as a potential countermeasure against neglected and emerging RNA viruses. Antiviral Res. 2018;153:85-94.
- [Google Scholar]
- Rationale of a loading dose initiation for hydroxychloroquine treatment in COVID-19 infection in the DisCoVeRy trial. J Antimicrob Chemother 2020 (e-pub ahead of print)
- [CrossRef] [Google Scholar]
- ClinicalTrials. Trial of Treatments for COVID-19 in Hospitalized Adults (DisCoVeRy). Available at: https://clinicaltrials.gov/ct2/show/NCT04315948.
- ClinicalTrials. Treatments for COVID-19: Canadian Arm of the SOLIDARITY Trial (CATCO). Available at: https://clinicaltrials.gov/ct2/show/NCT04330690?cond=solidarity+trial&draw=2&rank=1.
- Combination therapy with lopinavir/ritonavir, ribavirin and interferon-α for Middle East respiratory syndrome. Antivir Ther. 2016;21(5):455-459.
- [Google Scholar]
- Treatment of Middle East Respiratory Syndrome with a combination of lopinavir-ritonavir and interferon-β1b (MIRACLE trial): study protocol for a randomized controlled trial. Trials. 2018;19(1):81.
- [Google Scholar]
- A Trial of lopinavir-ritonavir in adults hospitalized with severe Covid-19. N Engl J Med. 2020;382(19):1787-1799.
- [Google Scholar]
- The convalescent sera option for containing COVID-19. J Clin Invest. 2020;130(4):1545-1548.
- [Google Scholar]
- COVID-19 and treatment with NSAIDs and corticosteroids: should we be limiting their use in the clinical setting? Ecancermedicalscience. 2020;14:1023.
- [Google Scholar]
- Clinical evidence does not support corticosteroid treatment for 2019-nCoV lung injury. Lancet. 2020;395:473-475. (10223)
- [Google Scholar]
- Surviving Sepsis Campaign: guidelines on the management of critically ill adults with Coronavirus Disease 2019 (COVID-19) Intensive Care Med. 2020;46(5):854-887.
- [Google Scholar]
- Early short course corticosteroids in hospitalized patients with COVID-19. Clin Infect Dis 2020:ciaa601.
- [Google Scholar]
- Safety, tolerability, and immunogenicity of a recombinant adenovirus type-5 vectored COVID-19 vaccine: a dose-escalation, open-label, non-randomised, first-in-human trial. Lancet. 2020;395:1845-1854. (10240)
- [Google Scholar]
- Developing Covid-19 vaccines at pandemic speed. N Engl J Med. 2020;382(21):1969-1973.
- [Google Scholar]
- The COVID-19 vaccine development landscape. Nat Rev Drug Discov. 2020;19(5):305-306.
- [Google Scholar]